Hydrogeology, Chemical Weathering, and Soil Formation
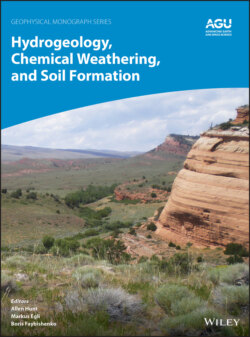
Реклама. ООО «ЛитРес», ИНН: 7719571260.
Оглавление
Allen Hunt. Hydrogeology, Chemical Weathering, and Soil Formation
Table of Contents
List of Tables
List of Illustrations
Guide
Pages
Geophysical Monograph Series
Hydrogeology, Chemical Weathering, and Soil Formation
DEDICATION
LIST OF CONTRIBUTORS
PREFACE
REFERENCES
1 Soil as a System: A History
ABSTRACT
1.1. INTRODUCTION
1.2. SOIL AS AN INDEPENDENT BODY
1.2.1. Dokuchaev’s Formula for Soil Formation
1.2.2. Shaw’s Elaboration
1.2.3. Jenny’s CLORPT Equation
1.3. SOIL AS A SYSTEM
1.3.1. Jenny’s Soil System
1.3.2. Simonson’s Soil System
1.3.3. Soil Energy System
1.4. SOIL AS A SPATIAL SYSTEM
1.4.1. Soil Profiles
1.4.2. Soil Catenas
1.4.3. Soil Landscapes
1.4.4. Soil‐Landscape Modeling
1.5. SOIL AS AN INTERDEPENDENT SYSTEM
1.5.1. The Critical Zone
1.5.2. New Pedologies
1.6. PROSPECT
ACKNOWLEDGMENTS
REFERENCES
2 Soils, Chemical Weathering, and Climate Change in Earth History
ABSTRACT
2.1. INTRODUCTION. 2.1.1. Definition of Paleosols
2.1.2. Soil‐Forming Factors
2.1.3. Paleosols as Potential Records of “Paleo‐Critical Zones”?
2.1.4. Paleosol Taxonomy
2.2. PRECAMBRIAN EON (4.55–0.54 GA) 2.2.1. General History
2.2.1.1. Plate tectonic “styles”
2.2.1.2. Geology and sedimentary deposits
2.2.2. Paleosol Records of Atmospheric CO2
2.2.3. Paleosol Records of Atmospheric Oxygen
2.2.4. Neoproterozoic “Snowball Earth” Events, 570–680 Ma, and Paleosols
2.2.5. Primitive State of Terrestrial Biotic Conditions and Cryptic Preservation
2.3. PHANEROZOIC EON (540 MA TO PRESENT)
2.3.1. Early Paleozoic Era. 2.3.1.1. Early life on land
2.3.1.2. Timing and sequence of terrestrial colonization by plants
2.3.1.3. Origin of roots
2.3.1.4. Environments of colonization
2.3.1.5. Early animal advances within the terrestrial realm
2.3.2. Middle Paleozoic Paleosols and Interpretations of Early Forest Ecosystems. 2.3.2.1. The dawn of arborescence
2.3.2.2. Innovative new plant groups
2.3.2.3. Ramifications of the emergence of early forest ecosystems. 2.3.2.3.1 Drawdown of atmospheric pCO 2 and acceleration of rates of silicate weathering
2.3.2.3.2. Fuel source for wildfires
2.3.2.3.3. Oceanic anoxic events and biotic crises
2.3.2.3.4. Sequestration of organic C
2.3.3. Carboniferous Coal Swamps and Glaciations, Gondwana Supercontinent. 2.3.3.1. Coal and clastic swamp and fluvial‐deltaic environments
2.3.3.2. Important floristic components
2.3.3.3. Major floral patterns
2.3.3.4. Carboniferous climate patterns interpreted from mineral paleosols
2.3.4. End‐Permian Extinctions and Climate Changes, Pangea Supercontinent
2.4. MESOZOIC
2.4.1. Atmospheric Gases
2.4.2. Megamonsoon
2.4.3. Biota
2.4.4. Paleosols. 2.4.4.1. Triassic
2.4.4.2. Jurassic
2.4.4.3. Cretaceous
2.4.5. Weathering Rates
2.5. CENOZOIC
2.5.1. Plate Tectonics
2.5.2. Atmospheric Gases
2.5.3. Environments, Climates, and Weathering during the Cenozoic
2.5.3.1. Cretaceous‐Paleogene extinction event (66 Ma)
2.5.3.2. Paleocene and Eocene hothouse conditions. 2.5.3.2.1. Paleocene‐Eocene thermal maximum: Climate and biota
2.5.3.2.2. Paleocene‐Eocene thermal maximum: Weathering
2.5.3.2.3. Early Eocene climatic optimum and hyperthermals: Climate and biota
2.5.3.2.4. Early Eocene climatic optimum and hyperthermals: Weathering
2.5.3.3. Eocene‐Oligocene transition into the icehouse world. 2.5.3.3.1. Eocene‐Oligocene transition: Climate and biota
2.5.3.3.2. Eocene‐Oligocene transition: Weathering
2.5.3.4. Miocene Epoch and the expansion of grasslands. 2.5.3.4.1. Miocene Epoch: Climate and biota
2.5.3.4.2. Miocene Epoch: Weathering
2.5.3.5. Pliocene‐Pleistocene glaciations
2.5.3.6. Late Pleistocene and Holocene transition: The Younger Dryas
2.5.3.7. Anthropocene
2.5.3.7.1. Anthropogenic effects on climate and biota
2.5.3.7.2. Anthropogenic effects on weathering
2.6. SUMMARY AND CONCLUSIONS. 2.6.1. Climate, Terrestrial Biota, and Weathering Are Inextricably Linked
2.6.2. Paleosols Are Important for Inferring Changes in Climate, Terrestrial Biota, and Weathering Over Time
ACKNOWLEDGMENTS
REFERENCES
3 Soil Formation, Vegetation Growth, and Water Balance: A Theory for Budyko
ABSTRACT
3.1. INTRODUCTION. 3.1.1. Water Partitioning at the Terrestrial Earth Surface
3.1.2. Water and Energy Conservation Foundation: The Budyko Approach
3.1.3. Fundamental Relationships Between ET and ET0: Climate Influences
3.2. HYPOTHESIS
3.3. THEORETICAL APPROACH AND STEADY‐STATE RESTRICTIONS
3.3.1. Percolation Theory, Solute Transport, and Chemical Weathering: Relevance to Soil Formation
3.3.2. Vegetation Growth and Net Primary Productivity (NPP)
3.3.3. Optimization of NPP and Result for ET (P) for Steady‐State Soil Conditions
3.3.4. Optimization for the Case of Transient Soil Development
3.3.5. Predicted Variability in ET
3.3.6. Variation of ET(P) with Dryness Index
3.3.7. Qualitative Modeling of NPP Across Climate Zones
3.4. COMPARISONS WITH DATA. 3.4.1. Global Terrestrial Mean Value of ET
3.4.2. Variation in ET with Aridity Index
3.4.3. Variability in ET for Specific Aridity Index
3.4.4. Climate Dependence of NPP(ET)
3.5. DISCUSSION AND POTENTIAL STRATEGIES FOR IMPROVEMENT
3.6. CONCLUSIONS
ACKNOWLEDGMENTS
REFERENCES
4 Earthworms, Plants, and Soils
ABSTRACT
4.1. PLANTS AND EARTHWORMS ARE ECOSYSTEM ENGINEERS. 4.1.1. Why Are Plants and Earthworms Called Ecosystem Engineers?
4.1.2. Relationships Between Selected Soil Properties, Plants, and Earthworms. 4.1.2.1. Soil texture
4.1.2.2. Soil porosity
4.1.2.3. Soil nutrient balance
4.1.3. Hotspots, Hot Moments in the Soil: Rhizosphere and Drilosphere
4.1.3.1. Rhizosphere
4.1.3.2. Drilosphere
4.2. PLANTS AND EARTHWORMS ARE IMPLIED IN SOIL FORMATION
4.2.1. Rock and Mineral Weathering
4.2.1.1. Plants. 4.2.1.1.1. Mechanical weathering
4.2.1.1.2. Chemical weathering
4.2.1.2. Earthworms. 4.2.1.2.1. Mineral weathering
4.2.1.2.2. Calcium cycling
4.2.2. Soil Structure Formation, Stabilization, and Disintegration
4.2.2.1. Soil structure and biota
4.2.2.2. Plants and soil aggregation
4.2.2.2.1. Root penetration
4.2.2.2.2. Changes in soil water regime
4.2.2.2.3. Agglutination of particles and aggregates
4.2.2.2.4. Dead roots
4.2.2.2.5. Entanglement by root and fungal hyphae
4.2.2.3. Implication of plants in organic matter stabilization
4.2.2.4. Earthworms and soil aggregation
4.2.2.4.1. Mucus and gut processes
4.2.2.4.2. Cast production
4.2.2.4.3. Burrowing
4.2.2.4.4. Aggregate disintegration
4.2.3. Bioturbation due to Plant and Earthworm Activities: Impacts on Soil Differentiation and Development
4.2.3.1. Bioturbation by plants: Mechanisms
4.2.3.2. Bioturbation by plants: Effects on soil heterogeneity and soil development
4.2.3.3. Postuprooting pedogenesis
4.2.3.4. Bioturbation by earthworms: Mechanisms and rates
4.2.3.5. Bioturbation by earthworms and pedogenesis
4.3. PLANTS AND EARTHWORMS CONTRIBUTE TO SOIL ECOSYSTEM SERVICES
4.3.1. Provisioning Services
4.3.2. Regulating Services. 4.3.2.1. Health and soil fertility
4.3.2.2. Water infiltration
4.3.2.3. Tritrophic interactions
4.3.2.4. Pollution control
4.3.2.5. Climate warming mitigation
4.4. CONCLUSIONS AND FUTURE PROSPECTS
REFERENCES
5 Tephra for the Trees? Geochemical Constraints on Weathering and Tephra Inputs to Soils on New Zealand’s North Island
ABSTRACT
5.1. INTRODUCTION
5.1.1. Conceptual Framework and Previous Work
5.2. FIELD SITE
5.3. METHODS
5.4. RESULTS. 5.4.1. Cosmogenic Nuclides and Soil Production Rate
5.4.2. Bedrock, Saprolite, and Soil Geochemistry
5.4.3. Possible Dust Inputs
5.5. FORWARD MODEL AND MODEL RESULTS
5.6. DISCUSSION. 5.6.1. Weathering Trends
5.6.2. Local History of Tephra Deposition, Soil Mixing, and Deforestation
5.6.3. Implications for Deep Weathering and Nutrient Cycling
5.7. CONCLUSIONS
ACKNOWLEDGMENTS
REFERENCES
6 The Origin and Formation of Clay Minerals in Alpine Soils
ABSTRACT
6.1. THE ORIGIN OF CLAYS IN SOILS. 6.1.1. Introduction
6.1.2. Inheritance, Transformation, and Neoformation
6.2. ENVIRONMENTAL CONDITIONS AND CLAY FORMATION. 6.2.1. Introduction
6.2.2. Time (Chronosequences)
6.2.3. Climate and Vegetation (Climosequences)
6.2.4. Substratum (Lithosequences)
6.2.5. Water Percolation and Erosion
6.3. CONCLUSION
ACKNOWLEDGEMENTS
REFERENCES
7 Weathering Rinds as Tools for Constraining Reaction Kinetics and Duration of Weathering at the Clast‐Scale
ABSTRACT
7.1. INTRODUCTION
7.2. WEATHERING RINDS AS TOOLS FOR CALIBRATED AGE DATING
7.3. CASE STUDIES
7.3.1. The Costa Rican Chronosequence
7.3.2. Nasuno‐ga‐hara Chronosequence, Central Japan
7.3.3. Basse‐Terre Island, Guadeloupe
7.4. VARIABLES INFLUENCING WEATHERING RIND THICKNESS
7.5. THE CORE‐RIND BOUNDARY AND INCIPIENT CHEMICAL WEATHERING
7.6. CHEMICAL AND MINERALOGIC PROPERTIES. 7.6.1. Compositional Variability
7.6.2. Mineralogic Gradients and Weathering Reactions
7.6.3. Open System Mass‐Balance Calculations
7.7. PHYSICAL AND MECHANICAL PROPERTIES ACROSS THE CORE‐RIND BOUNDARY. 7.7.1. Quantifying Changes in Color
7.7.2. Hardness Measurements
7.7.3. Porosity Development
7.8. DIFFUSION MODEL OF WEATHERING RIND DEVELOPMENT
7.9. QUANTIFYING THE DURATION OF WEATHERING
7.10. WEATHERING ADVANCE RATES ACROSS PRECIPITATION GRADIENTS
7.11. SUMMARY POINTS
ACKNOWLEDGMENTS
REFERENCES
8 Unraveling Loess Records of Climate Change from the Chinese Loess Plateau Using Process‐Based Models
ABSTRACT
8.1. INTRODUCTION
8.2. GLOBAL‐CHANGE RESPONSIVE MODELS OF SOIL FORMATION
8.2.1. Reconstruction of the Formation of Paleosols: Key Soil Model Output Variables
8.2.2. Prediction of Future Soil Development: Key Soil Model Output Variables and Processes
8.2.2.1. Soil as a natural capital
8.2.2.2. Soil as an ecosystem service
8.2.3. Model Responsiveness to Factors of Global Change
8.3. SOILGEN‐LOVECLIM: A SUITABLE COMBINATION FOR GLOBAL CHANGE STUDIES IN LOESS SOILS
8.3.1. LOVECLIM
8.3.2. SoilGen
8.3.3. Satisfying Data Needs Across Scales
8.3.4. Case Study: Comparison of Paleosols from MIS 5e and MIS 13
8.3.5. Case Study: Evolution of the Natural Capital and Ecosystem Services During MIS 5e
8.4. ACHIEVEMENTS AND CHALLENGES
REFERENCES
9 Relations Between Soil Development and Landslides
ABSTRACT
9.1. INTRODUCTION
9.2. HOW SOILS INCREASE SUSCEPTIBILITY TO LANDSLIDES
9.3. SOIL DEVELOPMENT
9.4. HOW LANDSLIDES AFFECT SOIL DEVELOPMENT
9.5. SOIL‐LANDSLIDE FEEDBACKS AND LANDSLIDE PATH‐DEPENDENCE
9.6. CONCLUSIONS
REFERENCES
10A Soils in Agricultural Engineering: Effect of Land‐Use Management Systems on Mechanical Soil Processes
ABSTRACT
10A.1. INTRODUCTION
10A.2. MECHANICAL PROCESSES IN SOILS
10A.2.1. Mechanical Stresses
10A.2.2. Time‐Dependent Strain Processes
10A.2.3. Stress Distribution in Soils
10A.2.4. Definition of Site‐Specific Soil Management
10A.3. EFFECT OF STRESS APPLICATION ON CHANGES IN ECOLOGICAL SOIL FUNCTIONS
10A.3.1. Capacity or Intensity Parameters
10A.3.2. Interaction Between Mechanical and Hydraulic Properties
10A.3.3. Coupling of Physical, Chemical, and Biological Processes
10A.4. CONCLUSIONS
REFERENCES
10B Soil Strength and Carbon Sequestration
ABSTRACT
10B.1. INTRODUCTION
10B.2. PROCESSES OF SOIL CARBON SEQUESTRATION
10B.3. SEQUESTRATION OF SOIL INORGANIC CARBON
10B.4. RESTORING SOIL PROPERTIES THROUGH ECO‐EFFECTIVE MANAGEMENT
10B.5. TECHNICAL AND ACTUAL RATE OF SOIL CARBON SEQUESTRATION
10B.6. SUSTAINABLE DEVELOPMENT GOALS OF THE UNITED NATIONS
10B.7. CONCLUSIONS
REFERENCES
11 Chemical Weathering in the McMurdo Dry Valleys, Antarctica
ABSTRACT
11.1. INTRODUCTION
11.2. STUDY AREA
11.3. FLUVIAL EROSION/DENUDATION
11.4. SOILS
11.5. SHALLOW GROUNDWATER SEEPS AND WATER TRACKS
11.6. STREAMS
11.7. WEATHERING PROCESSES. 11.7.1. Other Soil Processes/Cation Exchange and Authigenic CaCo3 Production‐Dissolution
11.7.2. Aluminosilicate Weathering by Shallow Groundwaters
11.7.3. Weathering in Streambeds
11.8. IMPLICATIONS OF MELTING BURIED ICE
11.9. CONCLUSIONS
ACKNOWLEDGMENTS
REFERENCES
12 Carbon and Nutrient Fluxes Within Southeastern Piedmont Critical Zones
ABSTRACT
12.1. INTRODUCTION
12.1.1. Geographic Setting
12.1.2. Conceptual Model Definition
12.2. METHODS. 12.2.1. Calhoun CZO
12.2.2. Lake Lanier
12.3. RESULTS. 12.3.1. Calhoun CZO
12.3.2. Lake Lanier
12.4. CONCLUSIONS
REFERENCES
13 Is This Steady State? Weathering and Critical Zone Architecture in Gordon Gulch, Colorado Front Range
ABSTRACT
13.1. INTRODUCTION
13.2. STUDY LOCATION
13.2.1. Gordon Gulch
13.2.2. Climate and Vegetation
13.2.3. Solar Radiation
13.3. CRITICAL ZONE ARCHITECTURE
13.3.1. Weathered Profile Thickness
13.3.2. Mobile Regolith
13.3.3. Methods of Determining Material Characteristics of the Critical Zone
13.3.3.1. Sample collection
13.3.3.2. Geochemistry
13.3.3.3. Rock quality
13.3.3.4. Rock strength
13.3.4. Critical Zone Geochemistry
13.3.5. Mechanical Properties of the Critical Zone
13.3.6. Residence Time in the Critical Zone
13.4. DISCUSSION
13.4.1. Is This Steady State?
13.5. SUMMARY
ACKNOWLEDGMENTS
REFERENCES
14 Where Are We and Where Are We Going? Pedogenesis Through Chemical Weathering, Hydrologic Fluxes, and Bioturbation
ABSTRACT
14.1. WHAT AND WHERE IS SOIL PHYSICALLY, HYDROLOGICALLY, CHEMICALLY, BIOLOGICALLY, CONCEPTUALLY, AND SCIENTIFICALLY?
14.1.1. Soil Formation, Erosion, and Burial
14.1.2. Biological Processes
14.2. SOIL‐FORMING FACTORS AND SOIL PRODUCTION; CONNECTIONS WITH GEOMORPHOLOGY AND HYDROLOGY
14.3. HUMAN IMPACTS AND RELEVANCE TO HUMAN SOCIETY
14.4. WHERE IS SOIL AS A DISCIPLINE?
14.5. RESEARCH DIRECTIONS, NETWORKS, AND FOUNDATIONAL SUPPORT: CURRENT AND FUTURE
14.6. WAYS FORWARD
REFERENCES
INDEX
WILEY END USER LICENSE AGREEMENT
Отрывок из книги
Geophysical Monograph 257
Allen Hunt Markus Egli Boris Faybishenko Editors
.....
where s is a soil property or the degree of pedogenesis, D is a set of dynamic vectors and dD/dt their rate of change through time, P is a set of passive vectors and dP/dt their rate of change through time. The dynamic vectors include energy fluxes, mass fluxes, the frequency of wetting and drying events, organisms, and pedoturbation. The passive vectors include parent material, the chemical environment of the soil, permanently low water tables, the stability of slopes, and pedogenetic accessions such as fragipans, natric horizons, and histic horizons.
Jonathan D. Phillips (1993a) explored the idea of progressive–regressive pedogenic changes in his own numerical model that incorporated relative rates of progressive and retrogressive pedogenesis and feedbacks between the rate and the degree of soil development:
.....