Introduction To Modern Planar Transmission Lines
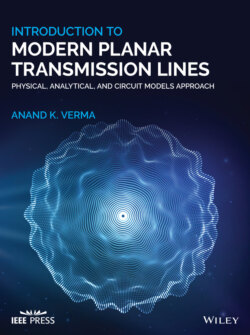
Реклама. ООО «ЛитРес», ИНН: 7719571260.
Оглавление
Anand K. Verma. Introduction To Modern Planar Transmission Lines
Table of Contents
List of Tables
List of Illustrations
Guide
Pages
Introduction to Modern Planar Transmission Lines. Physical, Analytical, and Circuit Models Approach
Preface
Acknowledgments
Author Biography
1 Overview of Transmission Lines: (Historical Perspective, Overview of Present Book) Introduction
Objectives
1.1 Overview of the Classical Transmission Lines
1.1.1 Telegraph Line
1.1.2 Development of Theoretical Concepts in EM‐Theory
Electrostatics and Scalar Potential
Magnetic Effect of Current
Ohm's Law
Electric Effect of the Time‐Varying Magnetic Field
Concept of the Magnetic Vector Potential
Maxwell's Dynamic Electromagnetic Theory
Generation and Transmission of Electromagnetic Waves
Further Information on Potentials
EM‐Modeling of Medium
1.1.3 Development of the Transmission Line Equations. Kelvin's Cable Theory
Heaviside Transmission Line Equation
1.1.4 Waveguides as Propagation Medium
1.2 Planar Transmission Lines
1.2.1 Development of Planar Transmission Lines
1.2.2 Analytical Methods Applied to Planar Transmission Lines
1.3 Overview of Present Book
1.3.1 The Organization of Chapters in This Book
Introductory Transmission Line and EM‐Wave Theory
Basic Planar Lines and Resonators
Analytical Methods
Contemporary Engineered Planar Structures
1.3.2 Key Features, Intended Audience, and Some Suggestions. Key Features
Intended Audience
Some Suggestions
References. Books
Journals
2 Waves on Transmission Lines – I: (Basic Equations, Multisection Transmission Lines) Introduction
Objectives
2.1 Uniform Transmission Lines
2.1.1 Wave Motion
2.1.2 Circuit Model of Transmission Line
The Resistance of a Line
The Inductance of a Line
The Capacitance of a Line
The Conductance of a Line
2.1.3 Kelvin–Heaviside Transmission Line Equations in Time Domain
The Loop Equation
The Node Equation
2.1.4 Kelvin–Heaviside Transmission Line Equations in Frequency‐Domain
2.1.5 Characteristic of Lossy Transmission Line
Characteristic Impedance
Propagation Constant
2.1.6 Wave Equation with Source
Shunt Current Source
The Loop Equation
The Node Equation
2.1.7 Solution of Voltage and Current‐Wave Equation
The Hyperbolic Form of a Solution
Short‐Circuited Receiving End
Open‐Circuited Receiving End
Matched and Mismatched Termination
Exponential Form of Solution
2.1.8 Application of Thevenin's Theorem to Transmission Line
Transfer Function
2.1.9 Power Relation on Transmission Line
Available Power from Generator
2.2 Multisection Transmission Lines and Source Excitation
2.2.1 Multisection Transmission Lines
2.2.2 Location of Sources
Current Source at the Junction of Finite Length Line and Infinite Length Line
Series Voltage Source
2.3 Nonuniform Transmission Lines
2.3.1 Wave Equation for Nonuniform Transmission Line
2.3.2 Lossless Exponential Transmission Line
References. Books
Journals
3 Waves on Transmission Lines – II: (Network Parameters, Wave Velocities, Loaded Lines) Introduction
Objectives
3.1 Matrix Description of Microwave Network
3.1.1 [Z] Parameters
Example 3.1
Solution
Example 3.2
Solution
3.1.2 Admittance Matrix
Example 3.3
Solution
Example 3.4
Solution
3.1.3 Transmission [ABCD] Parameter
Example 3.5
Solution
Example 3.6
Solution
Example 3.7
Solution
3.1.4 Scattering [S] Parameters
Basic Concept
Scattering [S] Matrix
Reflection Coefficient Sii
Transmission Coefficient Sij
Properties of [S] Matrix
Reciprocity Property
Unitary Property
Phase Shift Property
Example 3.8
Solution
Example 3.9
Solution
Example 3.10
Solution
Example 3.11
Solution
3.2 Conversion and Extraction of Parameters
3.2.1 Relation Between Matrix Parameters [Z] and [ABCD] Parameters
[S] and [Z] Parameters
[ABCD] and [S] Parameters
3.2.2 De‐Embedding of True S‐Parameters
3.2.3 Extraction of Propagation Characteristics
3.3 Wave Velocity on Transmission Line
3.3.1 Phase Velocity
3.3.2 Group Velocity
Formation of Two‐Frequency Wave‐Packet
3.4 Linear Dispersive Transmission Lines
3.4.1 Wave Equation of Dispersive Transmission Lines
3.4.2 Circuit Models of Dispersive Transmission Lines
Shunt Inductor Loaded Line
Backward Wave Supporting Line
Series Connected Parallel Lsh‐C Type Line
Series Capacitor Loaded LC‐Line
References. Books
Journals
4 Waves in Material Medium – I: (Waves in Isotropic and Anisotropic Media, Polarization of Waves) Introduction
Objectives
4.1 Basic Electrical Quantities and Parameters
4.1.1 Flux Field and Force Field
Gauss’s Law for Electric Flux
Gauss’s Law for Magnetic Flux
4.1.2 Constitutive Relations
4.1.3 Category of Materials. Dielectric Materials
Magnetic Materials
Conductors
4.2 Electrical Property of Medium
4.2.1 Linear and Nonlinear Medium
4.2.2 Homogeneous and Nonhomogeneous Medium
4.2.3 Isotropic and Anisotropic Medium
Symmetric Anisotropic Materials
Anti‐symmetric Anisotropic Materials
Gyroelectric Medium
Gyromagnetic Medium
Magneto‐dielectric Composite Materials
Magnetoelectric Materials
4.2.4 Nondispersive and Dispersive Medium
4.2.5 Non‐lossy and Lossy Medium
4.2.6 Static Conductivity of Materials
4.3 Circuit Model of Medium
4.3.1 RC Circuit Model of Lossy Dielectric Medium
4.3.2 Circuit Model of Lossy Magnetic Medium
4.4 Maxwell Equations and Power Relation
4.4.1 Maxwell’s Equations
4.4.2 Power and Energy Relation from Maxwell Equations
4.5 EM‐waves in Unbounded Isotropic Medium
4.5.1 EM‐wave Equation
4.5.2 1D Wave Equation
4.5.3 Uniform Plane Waves in Linear Lossless Homogeneous Isotropic Medium
4.5.4 Vector Algebraic Form of Maxwell Equations
4.5.5 Uniform Plane Waves in Lossy Conducting Medium
4.6 Polarization of EM‐waves
4.6.1 Linear Polarization
4.6.2 Circular Polarization
4.6.3 Elliptical Polarization
4.6.4 Jones Matrix Description of Polarization States
Jones Vector
Jones Matrix
Jones Matrix of Linear Polarizer
Jones Matrix of a Linear Polarizer Rotated at Angle θ with the y‐Axis
Transformation of E‐vector Components
Transformation of Jones Matrix of Polarizer
Jones Matrix for Retarder (Phase Shifter)
Jones Matrix of Half‐waveplate
Jones Matrix of Quarter‐waveplate
4.7 EM‐waves Propagation in Unbounded Anisotropic Medium
4.7.1 Wave Propagation in Uniaxial Medium
Waveplates and Phase Shifters
4.7.2 Wave Propagation in Uniaxial Gyroelectric Medium
4.7.3 Dispersion Relations in Biaxial Medium
4.7.4 Concept of Isofrequency Contours and Isofrequency Surfaces
4.7.5 Dispersion Relations in Uniaxial Medium
References. Books
Journals
5 Waves in Material Medium‐II: (Reflection & Transmission of Waves, Introduction to Metamaterials) Introduction
Objectives
5.1 EM‐Waves at Interface of Two Different Media
5.1.1 Normal Incidence of Plane Waves
5.1.2 The Interface of a Dielectric and Perfect Conductor
5.1.3 Transmission Line Model of the Composite Medium
5.2 Oblique Incidence of Plane Waves
5.2.1 TE (Perpendicular) Polarization Case
The Oblique Incidence on a Perfect Electric Conductor
5.2.2 TM (Parallel) Polarization Case
The Oblique Incidence on a Perfect Electric Conductor
5.2.3 Dispersion Diagrams of Refracted Waves in Isotropic and Uniaxial Anisotropic Media
5.2.4 Wave Impedance and Equivalent Transmission Line Model
Formulation of Transmission Line Model
Correspondence between Wave Impedance and Characteristic Impedance
Refection/Transmission Coefficients at Media Interface and Lines Junction
Computation of Reflection and Transmission Coefficients
5.3 Special Cases of Angle of Incidence
5.3.1 Brewster Angle
TE‐Polarization
TM‐Polarization
5.3.2 Critical Angle
Case #1: θ1< θc
Case#2: θ1 = θc
Case#3: θ1> θc
TE Polarization
TM Polarization
5.4 EM‐Waves Incident at Dielectric Slab
5.4.1 Oblique Incidence
5.4.2 Normal Incidence
5.5 EM‐Waves in Metamaterials Medium
5.5.1 General Introduction of Metamaterials and Their Classifications
5.5.2 EM‐Waves in DNG Medium
Lossless DNG Medium
Refractive Index of DNG Medium
Wave Impedance of DNG and SNG Media
Negative Refraction in DPS‐DNG Composite Medium
5.5.3 Basic Transmission Line Model of the DNG Medium
5.5.4 Lossy DPS and DNG Media
5.5.5 Wave Propagation in DNG Slab
Phase – Compensation in the DPS‐DNG Slab
Amplitude‐Compensation in the DNG Slab
5.5.6 DNG Flat Lens and Superlens
Veselago Flat Lens
Pendry Superlens Lens
HyperLens
5.5.7 Doppler and Cerenkov Radiation in DNG Medium
Doppler Effect
Inverse Doppler Effect
Cerenkov Radiation
Inverse Cerenkov Radiation
5.5.8 Metamaterial Perfect Absorber (MPA)
Salisbury Absorber
Metasurface Absorber
DNG Slab Absorber
Lossy DNG Slab with Conductor Backing
Lossy DNG Slab Without Conductor Backing
References. Books
Journals
6 Electrical Properties of Dielectric Medium. Introduction
Objectives
6.1 Modeling of Dielectric‐Medium
6.1.1 Dielectric Polarization
6.1.1.1 Electronic Polarization
6.1.1.2 Ionic Polarization
6.1.1.3 Orientational (Dipolar) Polarization
6.1.1.4 Space Charge (Interfacial) Polarization
6.1.2 Susceptibility, Relative Permittivity, and Clausius–Mossotti Model
6.1.2.1 Clausius–Mossotti Model
6.1.2.2 Lorentz Local Electric Field Model
6.1.3 Models of Polarizability
6.1.3.1 Electronic Polarizability
6.1.3.2 Ionic Polarizability
6.1.3.3 Orientational (Dipolar) Polarizability
6.1.4 Magnetization of Materials
6.2 Static Dielectric Constants of Materials
6.2.1 Natural Dielectric Materials
6.2.1.1 Nonpolar Solid
6.2.1.2 Polar Solid
6.2.2 Artificial Dielectric Materials
6.2.2.1 Classical Examples of Artificial Dielectrics
6.2.2.2 Computation of Dielectric Constant of Artificial Dielectrics
6.3 Dielectric Mixtures
6.3.1 General Description of Dielectric Mixture Medium
6.3.2 Limiting Values of Equivalent Relative Permittivity
6.3.2.1 Parallel Capacitors Model
6.3.2.2 Series Capacitors Model
6.3.2.3 Variational Model of Bounds on the Equivalent Relative Permittivity of the Mixture
6.3.3 Additional Equivalent Permittivity Models of Mixture
6.3.3.1 Maxwell Garnett Formula
6.3.3.2 Sihvola Formula
6.3.3.3 Bruggeman Formula
6.4 Frequency Response of Dielectric Materials
6.4.1 Relaxation in Material and Decay Law. 6.4.1.1 Relaxation in Conducting Medium
6.4.2 Polarization Law of Linear Dielectric‐Medium
6.4.2.1 Polarization Decay Law
6.4.2.2 Polarization Rise Law
6.4.3 Debye Dispersion Relation
6.4.3.1 Debye’s Dispersion Equation for Three Kinds of Polarizations
6.5 Resonance Response of the Dielectric‐Medium
6.5.1 Lorentz Oscillator Model
6.5.1.1 Low‐Frequency Relative Permittivity
6.5.1.2 Relative Permittivity at and Near the Resonance Frequency
6.5.1.3 High‐Frequency Relative Permittivity Above Resonance
6.5.2 Drude Model of Conductor and Plasma
6.5.3 Dispersion Models of Dielectric Mixture Medium
6.5.3.1 Maxwell Garnett–Debye Model
6.5.3.2 Maxwell Garnett–Lorentz Model
6.5.3.3 Maxwell Garnett–Drude Model
6.5.4 Kramers–Kronig Relation
6.6 Interfacial Polarization
6.6.1 Interfacial Polarization in Two‐Layered Capacitor Medium
6.6.1.1 Boundary Conditions
6.6.1.2 Steady‐State Condition
6.6.1.3 Transient Condition
6.7 Circuit Models of Dielectric Materials
6.7.1 Series RC Circuit Model
6.7.2 Parallel RC Circuit Model
6.7.3 Parallel Series Combined Circuit Model
6.7.4 Series Combination of RC Parallel Circuit
6.7.4.1 Lossless Upper Layer
6.7.4.2 Limiting Cases
6.7.4.3 Lossy Upper Layer
6.7.4.4 Equivalent Relative Permittivity
6.7.5 Series RLC Resonant Circuit Model
6.8 Substrate Materials for Microwave Planar Technology
6.8.1 Evaluation of Parameters of Single‐Term Debye and Lorentz Models. 6.8.1.1 Single‐Term Debye Model
6.8.1.2 Single‐term Lorentz Model
6.8.2 Multiterm and Wideband Debye Models
6.8.2.1 Multiterm Debye Model
6.8.2.2 Wideband Debye Model
6.8.3 Metasubstrates
References. Books
Journals
7 Waves in Waveguide Medium. Introduction
Objectives
7.1 Classification of EM‐Fields
7.1.1 Maxwell Equations and Vector Potentials
7.1.2 Magnetic Vector Potential
7.1.3 Electric Vector Potential
7.1.4 Generation of EM‐Field by Electric and Magnetic Vector Potentials
TMz Mode
TEz Mode
Hybrid Mode
For TEy Mode [LSEy Mode]
For TMy Mode [LSMy Mode]
7.2 Boundary Surface and Boundary Conditions
7.2.1 Perfect Electric Conductor (PEC)
Scalar Electric Potential
Scalar Magnetic Potential
7.2.2 Perfect Magnetic Conductor (PMC)
Scalar Electric Potential
Scalar Magnetic Potential
7.2.3 Interface of Two Media
7.3 TEM‐Mode Parallel‐Plate Waveguide
7.3.1 TEM Field in Parallel‐Plate Waveguide
7.3.2 Circuit Relations
7.3.3 Kelvin–Heaviside Transmission Line Equations from Maxwell Equations
7.4 Rectangular Waveguides
7.4.1 Rectangular Waveguide with Four EWs
TEz Modes
Eigenvalue and Eigenfunction
TEz Modal Fields
TMz Modes
Eigenvalue and Eigenfunction
TMz Modal Fields
Characteristics of Modes
Modal Propagation Constant
Wave Velocities
Modal Wave Impedance
Modal Fields
Surface Current on the Waveguide
EM‐wave Power Transfer in Waveguide
Mode
Mode
Attenuation
Conductor Loss
Dielectric Loss
7.4.2 Rectangular Waveguide with Four MWs
TEz Mode
TMz Mode
7.4.3 Rectangular Waveguide with Composite Electric and MWs
TEz Mode
TMz Mode
Current Distribution on the Bottom and Top Conductors
TEz Mode
TMz Mode
7.5 Conductor Backed Dielectric Sheet Surface Wave Waveguide
7.5.1 TMz Surface Wave Mode
7.5.2 TEz Surface Wave Mode
7.6 Equivalent Circuit Model of Waveguide
7.6.1 Relation Between Wave Impedance and Characteristic Impedance
7.6.2 Transmission Line Model of Waveguide
7.7 Transverse Resonance Method (TRM)
7.7.1 Standard Rectangular Waveguide
7.7.2 Dielectric Loaded Waveguide
TEy (LSEy) Hybrid Mode
TMy (LSMy) Hybrid Mode
7.7.3 Slab Waveguide
TEz Even Mode
TEz Odd Mode
TMz Even Mode
TMz Odd Mode
7.7.4 Conductor Backed Multilayer Dielectric Sheet
7.8 Substrate Integrated Waveguide (SIW)
7.8.1 Complete Mode Substrate Integrated Waveguide (SIW)
7.8.2 Half‐Mode Substrate Integrated Waveguide (SIW)
Model‐I, Radiation Model
Model‐II, Equivalent Waveguide Model
References. Books
Journals
8 Microstrip Line: Basic Characteristics. Introduction
Objectives
8.1 General Description
8.1.1 Conceptual Evolution of Microstrip Lines
8.1.2 Non‐TEM Nature of Microstrip Line
8.1.3 Quasi‐TEM Mode of Microstrip Line
8.1.4 Basic Parameters of Microstrip Line
8.2 Static Closed‐Form Models of Microstrip Line
8.2.1 Homogeneous Medium Model of Microstrip Line (Wheeler’s Transformation)
8.2.2 Static Characteristic Impedance of Microstrip Line
8.2.3 Results on Static Parameters of Microstrip Line
8.2.4 Effect of Conductor Thickness on Static Parameters of Microstrip Line
8.2.5 Effect of Shield on Static Parameters of Microstrip Line
8.2.6 Microstrip Line on Anisotropic Substrate
8.3 Dispersion in Microstrip Line
8.3.1 Nature of Dispersion in Microstrip
8.3.2 Waveguide Model of Microstrip
Frequency‐Dependent Equivalent Width
Frequency‐Dependent Characteristic Impedance
Higher‐Order Mode
8.3.3 Logistic Dispersion Model of Microstrip (Dispersion Law of Microstrip)
Correction Factor in LDM
8.3.4 Kirschning–Jansen Dispersion Model
8.3.5 Improved Model of Frequency‐Dependent Characteristic Impedance
8.3.6 Synthesis of Microstrip Line
8.4 Losses in Microstrip Line
8.4.1 Dielectric Loss in Microstrip
EM‐Wave in a Lossy Dielectric‐Medium
Representation of Lossy Dielectric‐Medium by a Lossy Capacitor
The Dielectric Loss of EM‐Wave in a Homogeneous Lossy Dielectric‐Medium
The Dielectric Loss of a Microstrip Line
8.4.2 Conductor Loss in Microstrip
Waveguide Model for Computation of Conductor Loss of Microstrip Line
Perturbation Method Using Surface Current with Edge Singularity
Conformal Mapping Method
Perturbation Method
Surface Impedance of Composite Layers Conductor
Wheeler’s Incremental Inductance Rule
8.5 Circuit Model of Lossy Microstrip Line
References. Books
Journals
9 Coplanar Waveguide and Coplanar Stripline: Basic Characteristics. Introduction
Objectives
9.1 General Description
9.2 Fundamentals of Conformal Mapping Method
9.2.1 Complex Variable
9.2.2 Analytic Function
9.2.3 Properties of Conformal Transformation
9.2.4 Schwarz–Christoffel (SC) Transformation
9.2.5 Elliptic Sine Function
1st Form of the 1st Kind of the Elliptic Integral
2nd Form of the 1st Kind of the Elliptic Integral
Complete Elliptic Integral
Approximation for the Elliptic Integral
3rd Form of the 1st Kind of Elliptic Integral
9.3 Conformal Mapping Analysis of Coplanar Waveguide
9.3.1 Infinite Extent CPW
9.3.2 CPW on Finite Thickness Substrate and Infinite Ground Plane
9.3.3 CPW with Finite Ground Planes
Step #I
Step #II
9.3.4 Static Characteristics of CPW. Infinite Dielectric Thickness
Finite Dielectric Thickness
9.3.5 Top‐Shielded CPW
9.3.6 Conductor‐Backed CPW
9.4 Coplanar Stripline
9.4.1 Symmetrical CPS on Infinitely Thick Substrate
9.4.2 Asymmetrical CPS (ACPS) on Infinitely Thick Substrate
9.4.3 Symmetrical CPS on Finite Thickness Substrate
Alternate Conformal Mapping of the CPS Line on Finitely Thick Substrate
Step #1
Step #2
9.4.4 Asymmetrical CPW (ACPW) and Asymmetrical CPS (ACPS) on Finite Thickness Substrate
ACPW Line
Step #1
Step #2
ACPS Line
9.4.5 Asymmetric CPS Line with Infinitely Wide Ground Plane
9.4.6 CPS with Coplanar Ground Plane [CPS–CGP]
9.4.7 Discussion on Results for CPS
9.5 Effect of Conductor Thickness on Characteristics of CPW and CPS Structures
9.5.1 CPW Structure
9.5.2 CPS Structure
9.6 Modal Field and Dispersion of CPW and CPS Structures
9.6.1 Modal Field Structure of CPW
CPW on a Finite Substrate
Conductor‐Backed CPW (CB‐CPW)
9.6.2 Modal Field Structure of CPS
9.6.3 Closed‐Form Dispersion Model of CPW
CPW on a Finitely Thick Substrate
Improved Dispersion Model
Frequency‐Dependent Characteristic Impedance
9.6.4 Dispersion in CPS Line
Closed‐Form Dispersion Model of CPS Line
9.7 Losses in CPW and CPS Structures
9.7.1 Conductor Loss
Wheeler's Incremental Inductance Method
Modified Perturbation Method
CPW Structure
CPS Structure
9.7.2 Dielectric Loss
9.7.3 Substrate Radiation Loss
9.8 Circuit Models and Synthesis of CPW and CPS
9.8.1 Circuit Model
9.8.2 Synthesis of CPW
Synthesis Expression Set ‐ I (Known w/h‐Ratio)
Synthesis Expression Set ‐ II (Known s/h‐Ratio)
9.8.3 Synthesis of CPS
References. Books
Journals
10 Slot Line: Basic Characteristics. Introduction
Objectives
10.1 Slot Line Structures
10.1.1 Structures of the Open Slot Line
10.1.2 Shielded Slot Line Structures
10.2 Analysis and Modeling of Slot Line
10.2.1 Magnetic Current Model
10.3 Waveguide Model
10.3.1 Standard Slot Line
Step #1: Formation of Width (a) of the Equivalent Waveguide
Step # 2: Formation of Height (b) of the Equivalent Waveguide
Modes in Waveguide
Summary of Expressions used in Waveguide
TE‐modes:
TM‐modes:
Determination of Susceptances Bd and Ba for Case‐A
Computation of Input Admittance Yi,0 due to the TE10 Mode
Computation of Input Admittance Yi,n Due to Higher‐order Modes
TE Mode
TM Mode
Case B: Magnetic Wall at y = ±b/2
Slowing‐Factor
Characteristic Impedance
10.3.2 Sandwich Slot Line
10.3.3 Shielded Slot Line
Computation of Bd at z = 0 for Waveguide #1
Input Admittance Yi,0 of the TE10 Mode
Input Admittance Yi,n of Higher‐order Modes
for the TE1,2n Mode
Input Admittance
Computation Ba at z = 0 for Waveguide #2
10.3.4 Characteristics of Slot Line
10.4 Closed‐form Models
10.4.1 Conformal Mapping Method
Standard Slot Line
Sandwich Slot Line
The Composite Substrate Slot Line
10.4.2 Krowne Model
Slowing‐Factor λg/λ0
Characteristic Impedance
10.4.3 Integrated Model
References. Books
Journals
Appendix – I
Appendix – II
11 Coupled Transmission Lines: Basic Characteristics. Introduction
Objectives
11.1 Some Coupled Line Structures
11.2 Basic Concepts of Coupled Transmission Lines
11.2.1 Forward and Reverse Directional Coupling
11.2.2 Basic Definitions
11.3 Circuit Models of Coupling
11.3.1 Capacitive Coupling – Even and Odd Mode Basics
Even‐Mode Excitation
Odd‐Mode Excitation
11.3.2 Forms of Capacitive Coupling
Capacitance Matrix
Direct Capacitances
Capacitive Coupling Coefficient
11.3.3 Forms of Inductive Coupling
Inductance Matrix
Direct Inductances
Inductive Coupling Coefficient
11.4 Even–Odd Mode Analysis of Symmetrical Coupled Lines
11.4.1 Analysis Method
S‐Parameters of Coupled Microstrip Lines
Input Impedance Matching
Isolation Between Ports
Coupled Strip Lines
11.4.2 Coupling Coefficients
11.5 Wave Equation for Coupled Transmission Lines
11.5.1 Kelvin–Heaviside Coupled Transmission Line Equations
11.5.2 Solution of Coupled Wave Equation
Case # 1: Asymmetrical Coupled Lines in the Inhomogeneous Medium
Case #2: Asymmetrical Coupled Lines in a Homogeneous Medium
Case # 3: Symmetrical Coupled Lines in an Inhomogeneous Medium
Case #4: Symmetrical Coupled Lines in the Homogeneous Medium
11.5.3 Modal Characteristic Impedance and Admittance
References. Books
Journals
12 Planar Coupled Transmission Lines. Introduction
Objectives
12.1 Line Parameters of Symmetric Edge Coupled Microstrips
12.1.1 Static Models for Even‐ and Odd‐Mode Relative Permittivity and Characteristic Impedances of Edge Coupled Microstrips
Even‐Mode Effective Relative Permittivity
Odd‐Mode Effective Relative Permittivity
Even‐Mode Characteristic Impedance
Odd‐Mode Characteristic Impedance
Effect of the Top Shield on Even‐Mode Characteristic Impedance
Effect of the Top Shield on Odd‐Mode Characteristic Impedance
Effect of Conductor Thickness on Microstrip
Another Simple Closed‐Form Expression
12.1.2 Frequency‐Dependent Models of Edge Coupled Microstrip Lines
Even‐Mode Effective Relative Permittivity
Even‐Mode Characteristics Impedance
Odd‐Mode Effective Relative Permittivity
Odd‐Mode Characteristics Impedance
12.2 Line Parameters of Asymmetric Coupled Microstrips
12.2.1 Static Parameters of Asymmetrically Coupled Microstrips
The Method Based on Symmetrically Coupled Microstrips
12.2.2 Frequency‐Dependent Line Parameters of Asymmetrically Coupled Microstrips
Tripathi Waveguides Dispersion Model
Extended Getsinger's Dispersion Models for C and π‐Modes
12.3 Line Parameters of Coupled CPW
12.3.1 Symmetric Edge Coupled CPW
Even‐Mode CPW Capacitance
Odd‐Mode CPW Capacitance
12.3.2 Shielded Broadside Coupled CPW
Odd Mode
Even Mode
12.4 Network Parameters of Coupled Line Section
12.4.1 Symmetrical Coupled Line in Homogeneous Medium
[Z] Parameters Description of 4‐Port Coupled Lines
Even‐Mode Excitation
Odd‐Mode Excitation
The 2‐Port Network of Coupled Transmission Lines
12.4.2 Symmetrical Coupled Microstrip Line in An Inhomogeneous Medium
[Z] Parameters
Even–odd Mode Excitation
Voltage Wave on a Line Section Excited by Current Sources at Both Ends
The Voltage on Conductor #a
The Voltage on Conductor #b
Y Parameters
Current Wave on a Line Section Excited by Voltage Sources at Both Ends
Even–Odd Mode Excitations
Current on Conductor #a
Current on Conductor #b
12.4.3 ABCD Matrix of Symmetrical Coupled Transmission Lines
12.5 Asymmetrical Coupled Lines Network Parameters
12.5.1 [ABCD] Parameters of the 4‐Port Network
Open Circuit Condition At Output Port [Ip3 = Ip4 = 0]
Determination of [A] Matrix
π‐Mode Voltage
C‐mode Voltage
Determination of [C] Matrix
Short‐Circuit Condition At Outputs (Vp4 = Vp3 = 0)
Determination of Elements of [B] Matrix
Determination of Elements of [D] Matrix
References. Books
Journals
13 Fabrication of Planar Transmission Lines. Introduction
Objectives
13.1 Elements of Hybrid MIC (HMIC) Technology
13.1.1 Substrates
13.1.2 Hybrid MIC Fabrication Process
13.1.3 Thin‐Film Process
Thermal Evaporation Process
Sputtering Process
13.1.4 Thick‐Film Process
Conventional Thick‐Film Technology
Photo‐Imageable Thick‐Film Technology
13.2 Elements of Monolithic MIC (MMIC) Technology
13.2.1 Fabrication Process
Epitaxial Growth Method
Mesa Etching Method
Ion Implantation Method
Isolation Implantation Method
13.2.2 Planar Transmission Lines in MMIC
Planar Transmission Lines for Passive Components and Interconnect
Si/GaAs‐Substrates
MIS/MOS Structure
Schottky Structure
Thin‐Film Structure
Flexible Polyethersulfone (PES) Substrate
Multilayer MMIC Lines
Buried Microstrip Line
Electrodes of Active Devices as Planar Transmission Lines
13.3 Micromachined Transmission Line Technology
13.3.1 MEMS Fabrication Process
Bulk Micromachining
Wet Etching
Dry Etching
Surface‐Micromachining
13.3.2 MEMS Transmission Line Structures
Membrane Technology
Microshield Technology
V‐Groove CMOS Technology
LIGA Technology
Waveguide Technology
13.4 Elements of LTCC
13.4.1 LTCC Materials and Process
13.4.2 LTCC Circuit Fabrication
13.4.3 LTCC Planar Transmission Line and Some Components
Microstrip
CPW to Microstrip Transition Structure
Coupled Microstrip Line
Branch‐line Coupler
Bandpass Filter
13.4.4 LTCC Waveguide and Cavity Resonators
References. Books
Journals
14 Static Variational Methods for Planar Transmission Lines. Introduction
Objectives
14.1 Variational Formulation of Transmission Line
14.1.1 Basic Concepts of Variation
14.1.2 Energy Method‐Based Variational Expression
Ex. 14.1
14.1.3 Green's Function Method‐Based Variational Expression
14.2 Variational Expression of Line Capacitance in Fourier Domain
14.2.1 Transformation of Poisson Equation in Fourier Domain
14.2.2 Transformation of Variational Expression of Line Capacitance in Fourier Domain
14.2.3 Fourier Transform of Some Charge Distribution Functions
Constant Charge Distribution
Linear Charge Distribution
Cubic Charge Distribution
Maxwell's Charge Distribution Function
Modified Maxwell's Distribution Function
Discrete Fourier Transform of ρi(x)
14.3 Analysis of Microstrip Line by Variational Method
14.3.1 Boxed Microstrip Line (Green's Function Method in Space Domain)
The Potential at the Central Conductor
Current Distribution on the Bottom and Top Ground Planes
14.3.2 Open Microstrip Line (Green's Function Method in Fourier Domain)
Conductor Thickness
14.3.3 Open Microstrip Line (Energy Method in Fourier Domain)
14.4 Analysis of Multilayer Microstrip Line
14.4.1 Space Domain Analysis of Multilayer Microstrip Structure
The TTL Method
Determination of the Variational Line Capacitance
Anisotropic Dielectric Layers
14.4.2 Static Spectral Domain Analysis of Multilayer Microstrip
14.5 Analysis of Coupled Microstrip Line in Multilayer Dielectric Medium
14.5.1 Space Domain Analysis
Case A: Multilayer Edge Coupled Microstrip Line
Case B: Multilayer Broadside Coupled Microstrip Line
14.5.2 Spectral Domain Analysis
14.6 Discrete Fourier Transform Method
14.6.1 Discrete Fourier Transform
Sine and Cosine Discrete Fourier Transforms
f(x) Odd Function
f(x) Even Function
Fourier Transform of the Derivatives
14.6.2 Boxed Microstrip Line
Determination of Green's Function in Discrete Fourier Domain
Line Capacitance Using Galerkin's Method
14.6.3 Boxed Coplanar Waveguide
One Term Basis Function
N‐Terms Basis Function
References. Books
Journals
15 Multilayer Planar Transmission Lines: SLR Formulation. Introduction
Objectives
15.1 SLR Process for Multilayer Microstrip Lines
15.1.1 SLR‐Process for Lossy Multilayer Microstrip Lines
15.1.2 Dispersion Model of Multilayer Microstrip Lines
SLR‐Dispersion Model
Improved SLR‐Dispersion Model
Shielded microstrip line
Suspended Microstrip Line
The Composite Substrate Microstrip Line
Case: εr2 > εr1
Case: εr1 > εr2
Shielded Composite Substrate Microstrip Line
Case: εr1 > εr2
Case: εr2 > εr1
Microstrip Line with Superstrate
15.1.3 Characteristic Impedance and Synthesis of Multilayer Microstrip Lines
15.1.4 Models of Losses in Multilayer Microstrip Lines
Dielectric Loss
Conductor Loss
15.1.5 Circuit Model of Multilayer Microstrip Lines
15.2 SLR Process for Multilayer Coupled Microstrip Lines
15.2.1 Equivalent Single‐Layer Substrate
Method – I: Common Equivalent Single‐layer Substrate
Method – II: Separate Equivalent Even‐/Odd‐Mode Single‐layer Substrate
15.2.2 Dispersion Model of Multilayer Coupled Microstrip Lines
15.2.3 Characteristic Impedance and Synthesis of Multilayer Coupled Microstrips
15.2.4 Loss Models of Multilayer Coupled Microstrip Lines
Dielectric Losses
Conductor Losses
15.3 SLR Process for Multilayer ACPW/CPW
15.3.1 Single‐Layer Reduction (SLR) Process for Multilayer ACPW/CPW
15.3.2 Static SDA of Multilayer ACPW/CPW Using Two‐Conductor Model
Elements of Dyadic Green's Function
Static Line Parameters of Multilayer ACPW/CPW
15.3.3 Dispersion Models of Multilayer ACPW/CPW
15.3.4 Loss Models of Multilayer ACPW/CPW
15.4 Further Consideration of SLR Formulation
References. Books
Journals
16 Dynamic Spectral Domain Analysis. Introduction
Objectives
16.1 General Discussion of SDA
16.2 Green's Function of Single‐Layer Planar Line
16.2.1 Formulation of Field Problem
16.2.2 Case #1: CPW and Microstrip Structures
Step #1: Application of Boundary Condition
Step #2: Application of Continuity Condition
Step #3: Assumption of the Slot‐Field
Determination of Strip Current Densities
Longitudinal Current Density Giving Green's Function Components
Transverse Current Density Giving Green's Function Components
Admittance Type Green's Function of CPW and Slotline
Impedance Type Green's Function of Microstrip
Determination of Z22
16.2.3 Case #II – Sides: MW – EW, Bottom: MW, Top: EW
Region #1 (Lower Region)
Region #2 (Upper Region)
The Tangential Field Components in Region #1
The Tangential Field Components in Region #2
16.3 Solution of Hybrid Mode Field Equations (Galerkin's Method in Fourier Domain)
16.3.1 Microstrip
15.2.1 One‐Term Basis Function for Longitudinal Current Jz(x)
One‐Term Basis Function for Both Transverse and Longitudinal Currents
16.3.2 CPW Structure
16.4 Basis Functions for Surface Current Density and Slot Field
16.4.1 Nature of the Field and Current Densities
16.4.2 Basis Functions and Nature of Hybrid Modes. Microstrip
Legendre Polynomials
Singular Sinusoidal Basis Functions
Singular Chebyshev Polynomial Basis Functions
Slotline
CPW Structure
Fourier Transform of Ezm(x) Even Mode
Fourier Transform of Ezm(x) Odd Mode
Fourier Transform of Exm(x) Even Mode
Fourier Transform of Exm(x) Odd Mode
16.5 Coplanar Multistrip Structure
16.5.1 Symmetrical Coupled Microstrip Line
16.6 Multilayer Planar Transmission Lines
16.6.1 Immittance Approach for Single‐Level Strip Conductors
TTL Equivalent Circuits for LSE and LSM Modes
TEy Mode
TMy Mode
Equivalent Transverse Transmission Line for LSE (TEy) Mode
Equivalent Transverse Transmission Line for LSM (TMy) Mode
Determination of the [Z] – Type Green's Function
Determination of [Y] Type Green's Function for Slot Line and CPW
16.6.2 Immittance Approach for Multilevel Strip Conductors
Determination of
Determination of
Determination of
References. Books
Journals
17 Lumped and Line Resonators: Basic Characteristics. Introduction
Objectives
17.1 Basic Resonating Structures
17.2 Zero‐Dimensional Lumped Resonator
17.2.1 Lumped Series Resonant Circuit
17.2.2 Lumped Parallel Resonant Circuit
17.2.3 Resonator with External Circuit
17.2.4 One‐Port Reflection‐Type Resonator
Reflection‐Type Series Resonator
Reflection‐Type Parallel Resonator
17.2.5 Two‐Port Transmission‐Type Resonator
Case‐1: Series‐Connected Series Resonant Circuit
Case‐ 2: Series‐Connected Parallel Resonant Circuit
Case‐3: Shunt‐Connected Parallel Resonator
Case‐4: Shunt‐Connected Series Resonance
17.2.6 Two‐Port Reaction‐Type Resonator
17.3 Transmission Line Resonator
17.3.1 Lumped Resonator Modeling of Transmission Line Resonator
Case‐I: Both Ends Short‐Circuited λg/2 Line
Case‐II: Short‐Circuited (λg/4) Transmission Line
Case‐III: Both Ends Open‐Circuited λg/2 Line
Case‐IV: Open‐Circuited λg/4 Line
17.3.2 Modal Description of λg/2 Short‐Circuited Line Resonator
References. Books
Journals
18 Planar Resonating Structures. Introduction
Objectives
18.1 Microstrip Line Resonator
18.1.1 λg/2 Open‐end Microstrip Resonator
18.1.2 λg/2 and λg/4 Short‐circuited Ends Microstrip Resonator
18.1.3 Microstrip Ring Resonator
Equivalent Circuit Model of Ring Resonator
18.1.4 Microstrip Step Impedance Resonator
Control of Spurious Harmonic Frequency
Optimum Resonant Length
18.1.5 Microstrip Hairpin Resonator
18.2 CPW Resonator
18.3 Slot Line Resonator
18.4 Coupling of Line Resonator to Source and Load
18.4.1 Direct‐coupled Resonator
Short‐circuited λg/2‐line Resonator
Short‐circuited λg/4‐line Resonator
18.4.2 Reactively Coupled Line Resonator
Inductive Coupling
Capacitive Coupling
18.4.3 Tapped Line Resonator
18.4.4 Feed to Planar Transmission Line Resonator
18.5 Coupled Resonators
18.5.1 Coupled Microstrip Line Resonator
18.5.2 Circuit Model of Coupled Microstrip Line Resonator
Electric (Capacitive) Coupling
Magnetic (Inductive) Coupling
Hybrid Coupling – Both Capacitive and Inductive Coupling
18.5.3 Some Structures of Coupled Microstrip Line Resonator
18.6 Microstrip Patch Resonators
18.6.1 Rectangular Patch
18.6.2 Modified Wolff Model (MWM)
18.6.3 Circular Patch
18.6.4 Ring Patch
18.6.5 Equilateral Triangular Patch
18.7 2D Fractal Resonators
18.7.1 Fractal Geometry
Similarity Dimension and Basic Characteristics of Fractal Structures
Koch Curves and Koch Islands
Minkowski Curves
Hilbert Curves
Sierpinski Triangles and Sierpinski Carpet. Sierpinski Triangles
Sierpinski Carpet
18.7.2 Fractal Resonator Antenna
18.7.3 Fractal Resonators
18.8 Dual‐Mode Resonators
18.8.1 Dual‐Mode Patch Resonators
Dual‐mode Square Patch Resonator
Dual‐mode Circular Patch Resonator
Dual‐mode Triangular Patch Resonator
18.8.2 Dual‐Mode Ring Resonators
References. Books
Journals
19 Planar Periodic Transmission Lines. Introduction
Objectives
19.1 1D and 2D Lattice Structures
19.1.1 Bragg's Law of Diffraction
19.1.2 Crystal Lattice Structures
Direct – Lattice
Reciprocal Lattice
1D Reciprocal Lattice
2D Reciprocal Lattice
2D Rectangular Lattice
2D Hexagonal Lattice
19.1.3 Concept of Brillouin Zone
1D Brillouin Zone
2D Brillouin Zone
19.2 Space Harmonics of Periodic Structures
19.2.1 Floquet–Bloch Theorem and Space Harmonics
19.3 Circuit Models of 1D Periodic Transmission Line
19.3.1 Periodically Loaded Artificial Lines
19.3.2 [ABCD] Parameters of Unit Cell
19.3.3 Dispersion in Periodic Lines
19.3.4 Characteristics of 1D Periodic Lines
Dispersion Characteristics. Loading Elements: Series Inductor/Shunt Capacitor
The Lower Edge of the Passband
The Upper Edge of the Passband
Loading Elements: Series Capacitor/Shunt Inductor
Loading Elements: Shunt Resonant Circuit/Series Resonant Circuit
Characteristics of Bloch Impedance
19.3.5 Some Loading Elements of 1D Periodic Lines
19.3.6 Realization of Planar Loading Elements
Realization of Capacitors in Microstrip
Realization of Inductors in Microstrip
Realization of Resonant Circuits in Microstrip
19.4 1D Planar EBG Structures
19.4.1 1D Microstrip EBG Line
Periodic Loading of Substrate
Periodic Loading of Ground Conductor
Periodic Loading and Variation of Strip Conductor
19.4.2 1D CPW EBG Line
Series Inductor Loading
Series Capacitor Loading
Shunt Capacitor Loading
Shunt Inductor Loading
References. Books
Journals
20 Planar Periodic Surfaces. Introduction
Objectives
20.1 2D Planar EBG Surfaces
20.1.1 General Introduction of EBG Surfaces
20.1.2 Characteristics of EBG Surface
The ω − β Dispersion Diagram
The Reflection Phase Diagram
20.1.3 Horizontal Wire Dipole Near EBG Surface
Anisotropic EBG Surface
EBG as a Reflecting Surface
EBG as a Ground Plane Surface
20.2 Circuit Models of Mushroom‐Type EBG
20.2.1 Basic Circuit Model
20.2.2 Dynamic Circuit Model
Grid Impedance of a Matrix of Patches
Dielectric Surface Impedance
Surface Impedance of EBG Surface
Reflection Coefficient and Dispersion Relation of EBG Surface
Dispersion Model of EBG Surface
Polarization‐Dependent Patch Capacitance
20.3 Uniplanar EBG Structures
20.4 2D Circuit Models of EBG Structures
20.4.1 Shunt‐Connected 2D Planar EBG Circuit Model
Bloch Impedance
Special Case – Uniplanar 2D EBG Structure
20.4.2 Series‐Connected 2D Planar EBG Circuit Model
References. Books
Journals
21 Metamaterials Realization and Circuit Models – I: (Basic Structural Elements and Bulk Metamaterials) Introduction
Objectives
21.1 Artificial Electric Medium
21.1.1 Polarization in the Wire Medium
21.1.2 Equivalent Parallel Plate Waveguide Model of Wire Medium
21.1.3 Reactance Loaded Wire Medium
Inductively Loaded Wire Medium
Capacitively Loaded Wire Medium
21.2 Artificial Magnetic Medium
21.2.1 Characteristics of the SRR
21.2.2 Circuit Model of the SRR
Computation of Effective Relative Permeability of Loop Medium
21.2.3 Computation of Equivalent Circuit Parameters of SRR
21.2.4 Bi‐anisotropy in the SRR Medium
21.2.5 Variations in SRR Structure
21.3 Double Negative Metamaterials
21.3.1 Composite Permittivity–Permeability Functions
Drude–Lorentz Function
Lorentz–Lorentz Function
21.3.2 Realization of Composite DNG Metamaterials
Strip Wire and SRR (SW‐SRR) Composite Element
CLS–SRR Composite Element
CLS–CLL Composite Element
21.3.3 Realization of Single‐Structure DNG Metamaterials
Ω‐Particle Metamaterials
S‐ring Particle Metamaterials
21.4 Homogenization and Parameters Extraction
21.4.1 Nicolson–Ross–Weir (NRW) Method
Computation of Relative Permittivity and Permeability of the Slab
NRW Method
The Relation between S‐parameters and Reflection–Transmission Coefficients
Extraction of Refractive Index and Intrinsic Impedance of Equivalent Homogeneous Slab
Alternate Sets of Expressions for Parameters Retrieval
Resolving Branching Problem
Using Two Thicknesses of a Slab
Iterative Method
Using Kramers–Kronig Relations
21.4.2 Dynamic Maxwell Garnett Model
Mie Scattering
Dynamic Maxwell Garnett Model # I
Dynamic Maxwell Garnett Model # II
Equivalent Mie Magnetic Resonator of SRR Inclusion
Equivalent RLC Circuit Model of Mie Magnetic Resonator
References. Books
Journals
22 Metamaterials Realization and Circuit Models – II: (Metalines and Metasurfaces) Introduction
Objectives
22.1 Circuit Models of 1D‐Metamaterials
22.1.1 Homogenization of the 1D‐medium
22.1.2 Circuit Equivalence of Material Medium
DPS‐Medium (L–C) Line (L‐Series, C‐Shunt)
ENG‐Medium (L–L) Line (L‐Series, L‐Shunt)
DNG‐Medium (C–L) Line (C‐Series, L‐Shunt)
MNG‐Medium (C–C) Line (C‐Series, C‐Shunt)
22.1.3 Single Reactive Loading of Host Medium
Series Capacitance in the Series Arm
Parallel Inductance in the Shunt Arm
Parallel Capacitance in the Series Arm
Series Inductance in the Shunt Arm
22.1.4 Single Reactive Loading of Host Medium with Coupling
22.1.5 Circuit Models of 1D Metalines
Series–Parallel Configuration
Unbalanced CRLH Metaline
Region ω < ωmp < ωep
Region (ωep < ω < ωmp), or (ωmp < ω < ωep)
Region ω > ωep > ωmp
Balanced CRLH metalines
Parallel–Series Configuration
Parallel–Parallel Configuration
Series–Series Configuration
22.2 Nonresonant Microstrip Metalines
22.2.1 Series–Parallel (CRLH) Metalines
22.2.2 Cascaded MNG–ENG (CRLH) Metalines
22.2.3 Parallel–Series (D‐CRLH) Metalines
22.3 Resonant Metalines
22.3.1 Resonant Inclusions
22.3.2 Microstrip Resonant Metalines
CSRR‐Based Microstrip Metaline
SRR‐Based Microstrip Metaline
22.3.3 CPW‐Resonant Metalines
SRR‐Based CPW Metaline
CSRR‐Based CPW Metalines
22.4 Some Applications of Metalines
22.4.1 Backfire to Endfire Leaky Wave Antenna
22.4.2 Metaline‐Based Microstrip Directional Coupler
22.4.3 Multiband Metaline‐Based Components
Dual‐band Components
Quad‐band Components
22.5 Modeling and Characterization of Metsurfaces
22.5.1 Characterization of Metasurface
Schelkunoff's Equivalent Surface
Modeling of Metasurface
Stage – 1
Stage – 2
Stage – 3
Stage – 4
Stage – 5
Stage – 6
Anisotropic Metasurface
Bianisotropic Metasurface
22.5.2 Reflection and Transmission Coefficients of Isotropic Metasurfaces
Perfect Reflection
Perfect Transmission
Metasurface with only Electric Polarization
22.5.3 Phase Control of Metasurface
Controlling Size of Inclusions over a Spatial Period
Controlling Rotation of Inclusions over a Spatial Period
The Response of Rotated Anisotropic Inclusion
22.5.4 Generalized Snell's Laws of Metasurfaces
Anomalous Reflection Law
Anomalous Refraction Law
22.5.5 Surface Waves on Metasurface
Surface Waves on Uniform Metasurface
Surface Waves on Nonuniform Metasurfaces
22.6 Applications of Metasurfaces
22.6.1 Demonstration of Anomalous Reflection and Refraction of Metasurfaces
Reflection Type Metasurfaces
1D Phase‐gradient Metasurface
2D Phase Gradient Metasurface
Transmission Type Metasurfaces
22.6.2 Reflectionless Transmission of Metasurfaces
Huygens Metasurfaces
Metasurfaces Using Fabry–Perot Resonance‐Based Inclusions
Choice of Multilayered Inclusion
Puncharatnum–Berry Phase Control
22.6.3 Polarization Conversion of Incident Plane Wave
Reflection‐type Metasurfaces
Transmission‐type Metasurfaces
Quarter – Wave Plate
Beam Refracting Gradient Metasurface
Circularly Polarized Microstrip Patch Antenna on Metasurface Substrate
Circularly Polarized Slot Antenna Using Metasurface Superstrate
References. Books
Journals
Index. a
b
c
d
e
f
g
h
i
j
k
l
m
n
o
p
q
r
s
t
u
v
w
z
WILEY END USER LICENSE AGREEMENT
Отрывок из книги
Anand K. Verma
.....
The voltage and current in the phasor form are written as follows:
(2.1.108)
.....