Solid State Chemistry and its Applications
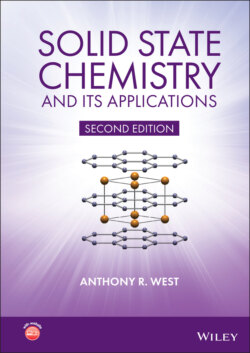
Реклама. ООО «ЛитРес», ИНН: 7719571260.
Оглавление
Anthony R. West. Solid State Chemistry and its Applications
Table of Contents
List of Tables
List of Illustrations
Guide
Pages
Solid State Chemistry and its Applications
Preface
Companion Website
Crystal Viewer
Crystal Structure Library
Biography
Solid State Chemistry, an Overview of the Discipline: Chemistry – Solid State Chemistry – Materials Chemistry – Materials Science and Engineering
Materials chemistry. Synthesis – structure determination – physical properties – new materials. Materials science. Processing and fabrication – characterisation – optimisation of properties and testing – improved/new materials for engineering applications in products or devices
1 Crystal Structures, Crystal Chemistry, Symmetry and Space Groups
1.1 Unit Cells and Crystal Systems
1.2 Symmetry. 1.2.1 Rotational symmetry; symmetry elements and operations
1.2.2 Quasicrystals
1.2.3 Mirror symmetry
1.2.4 Centre of symmetry and inversion axes
1.2.5 Point symmetry and space symmetry
1.3 Symmetry and Choice of Unit Cell
1.4 Lattice, Bravais Lattice
1.5 Lattice Planes and Miller Indices
1.6 Indices of Directions
1.7 d‐Spacing Formulae
1.8 Crystal Densities and Unit Cell Contents
1.9 Description of Crystal Structures
1.10 Close Packed Structures – Cubic and Hexagonal Close Packing
1.11 Relationship Between Cubic Close Packed and Face Centred Cubic
1.12 Hexagonal Unit Cell and Close Packing
1.13 Density of Close Packed Structures
1.14 Unit Cell Projections and Atomic Coordinates
1.15 Materials that can be Described as Close Packed. 1.15.1 Metals
1.15.2 Alloys
1.15.3 Ionic structures
1.15.3.1 Tetrahedral and octahedral sites
1.15.3.2 Relative sizes of tetrahedral and octahedral sites
1.15.3.3 Location of tetrahedral and octahedral sites in an fcc unit cell; bond length calculations
1.15.3.4 Description of crystal structures; fractional atomic coordinates
1.15.4 Covalent network structures
1.15.5 Molecular structures
1.15.6 Fullerenes and fullerides
1.16 Structures Built of Space‐Filling Polyhedra
1.17 Some Important Structure Types. 1.17.1 Rock salt (NaCl), zinc blende or sphalerite (ZnS), fluorite (CaF2), antifluorite (Na2O)
1.17.1.1 Rock salt structure
1.17.1.2 Zinc blende (sphalerite) structure
1.17.1.3 Antifluorite/fluorite structure
1.17.1.4 Cuprite structure, Cu2O
1.17.1.5 Bond length calculations
1.17.2 Diamond
1.17.3 Wurtzite (ZnS) and nickel arsenide (NiAs)
1.17.4 Caesium chloride (CsCl)
1.17.5 Other AX structures
1.17.6 Rutile (TiO2), cadmium iodide (CdI2), cadmium chloride (CdCl2) and caesium oxide (Cs2O)
1.17.7 Perovskite
1.17.7.1 Tolerance factor
1.17.7.2 BaTiO3
1.17.7.3 Tilted perovskites: Glazer notation
1.17.7.4 CaCu3Ti4O12, CCTO
1.17.7.5 Anion‐deficient perovskites
1.17.7.6 Stoichiometry–property relations
1.17.7.7 Cation‐ordered perovskites
1.17.7.8 Hybrid organic–inorganic halide perovskites
1.17.7.9 Anti‐perovskites
1.17.7.10 Mixed anion perovskites: oxynitrides and oxyfluorides
1.17.7.11 Hexagonal perovskites
1.17.8 Rhenium trioxide (ReO3), perovskite tungsten bronzes, tetragonal tungsten bronzes and tunnel structures
1.17.9 Spinel
1.17.10 Olivine
1.17.11 Corundum, ilmenite and LiNbO3
1.17.12 Fluorite‐related structures, pyrochlore, weberite and rare earth sesquioxides
1.17.13 Garnet
1.17.14 Perovskite‐rock salt intergrowth structures: K2NiF4, Ruddlesden–Popper, Aurivillius and Dion Jacobsen phases and layered cuprate superconductors
1.17.15 The aluminium diboride structure (AlB2)
1.17.16 Silicate structures – some tips to understanding them
1.18 Point Groups and Space Groups
1.18.1 Point groups
1.18.2 Stereographic projections and equivalent positions
1.18.3 Point symmetry of molecules: general and special positions
1.18.4 Centrosymmetric and non‐centrosymmetric point groups
1.18.5 Space groups
1.18.5.1 Triclinic P
1.18.5.2 Monoclinic C2
1.18.5.3 Monoclinic C2/m
1.18.5.4 Orthorhombic P2221
1.18.5.5 Orthorhombic F222
1.18.5.6 Tetragonal I41
1.18.6 Space groups and crystal structures
1.18.6.1 The perovskite structure, SrTiO3
1.18.6.2 The rutile structure, TiO2
1.18.7 Systematic absences in diffraction patterns and space groups
2 Crystal Defects, Non‐stoichiometry and Solid Solutions. 2.1 Perfect and Imperfect Crystals
2.2 Types of Defect: Point Defects
2.2.1 Schottky defect
2.2.2 Frenkel defect
2.2.2.1 The Kroger–Vink notation for crystal defects
2.2.2.2 Thermodynamics of Schottky and Frenkel defect formation
Schottky defects
Frenkel defects
2.2.3 Colour centres
2.2.4 Vacancies and interstitials in non‐stoichiometric crystals: extrinsic and intrinsic defects
2.2.5 Defect clusters or aggregates
2.2.6 Interchanged atoms: order–disorder phenomena
2.3 Solid Solutions of Ionic Materials
2.3.1 Substitutional solid solutions
2.3.2 Interstitial solid solutions
2.3.3 More complex solid solution mechanisms: aliovalent substitution
2.3.3.1 Ionic compensation mechanisms
2.3.3.2 Electronic compensation: metals, semi‐ and superconductors
2.3.4 Thermodynamically stable and metastable solid solutions
2.3.5 Experimental methods for studying solid solutions. 2.3.5.1 X‐ray powder diffraction, XRD
2.3.5.2 Density measurements
2.3.5.3 Changes in other properties – thermal activity and DTA/DSC
2.4 Extended Defects
2.4.1 Crystallographic shear structures
2.4.2 Stacking faults
2.4.3 Subgrain boundaries and antiphase domains (boundaries)
2.4.4 When do defects in a structure become a new structure?
2.5 Dislocations and Mechanical Properties of Solids
2.5.1 Edge dislocations
2.5.2 Screw dislocations
2.5.3 Dislocation loops
2.5.4 Dislocations and crystal structure
2.5.5 Mechanical properties of metals
2.5.6 Dislocations, vacancies and stacking faults
2.5.7 Dislocations and grain boundaries
3 Bonding in Solids. 3.1 Overview: Ionic, Covalent, Metallic, van der Waals and Hydrogen Bonding in Solids
3.2 Ionic Bonding
3.2.1 Ions and ionic radii
3.2.2 Ionic structures – general principles
3.2.3 The radius ratio rules
3.2.4 Borderline radius ratios and distorted structures
3.2.5 Lattice energy of ionic crystals
3.2.6 Kapustinskii's equation
3.2.7 The Born–Haber cycle and thermochemical calculations
3.2.8 Stabilities of real and hypothetical ionic compounds. 3.2.8.1 Inert gas compounds
3.2.8.2 Lower and higher valence compounds
3.2.9 Effect of partial covalent bonding on crystal structures
3.2.10 Effective nuclear charge
3.2.11 Electronegativity and partially charged atoms
3.2.12 Coordinated polymeric structures – Sanderson's model
3.2.13 Mooser–Pearson plots and ionicities
3.2.14 Bond valence and bond length
3.2.15 Non‐bonding electron effects
3.2.15.1 d‐electron effects
3.2.15.2 Inert pair effect
3.3 Covalent Bonding
3.3.1 Particle‐wave duality, atomic orbitals, wavefunctions and nodes
3.3.2 Orbital overlap, symmetry and molecular orbitals
3.3.3 Valence bond theory, electron pair repulsion, hybridisation and oxidation states
3.4 Metallic Bonding and Band Theory
3.4.1 Band structure of metals
3.4.2 Band structure of insulators
3.4.3 Band structure of semiconductors: silicon
3.4.4 Band structure of inorganic solids
3.4.4.1 III–V, II–VI and I–VII compounds
3.4.4.2 Transition metal compounds
3.4.4.3 Fullerenes and graphite
3.5 Bands or Bonds: A Final Comment
4 Synthesis, Processing and Fabrication Methods. 4.1 General Observations
4.2 Solid State Reaction or Shake ‘n Bake Methods
4.2.1 Nucleation and growth, epitaxy and topotaxy
4.2.2 Practical considerations and some examples of solid state reactions
4.2.2.1 Li4SiO4
4.2.2.2 YBa2Cu3O7−δ
4.2.2.3 Na β/β″ alumina
4.2.3 Combustion synthesis
4.2.4 Mechanochemical synthesis
4.3 Low Temperature or Chimie Douce Methods
4.3.1 Alkoxide sol–gel method
4.3.1.1 Synthesis of MgAl2O4
4.3.1.2 Synthesis of silica glass
4.3.1.3 Spinning of alumina fibres
4.3.1.4 Preparation of indium tin oxide (ITO) and other coatings
4.3.1.5 Fabrication of YSZ ceramics
4.3.2 Sol‐gel method using oxyhydroxides; solution chemistry of Al and Fe
4.3.2.1 Synthesis of zeolites
4.3.2.2 Preparation of alumina‐based abrasives and films
4.3.3 Citrate gel and Pechini processes
4.3.4 Use of homogeneous, single‐source precursors
4.3.5 Hydrothermal and solvothermal synthesis
4.3.6 Microwave synthesis
4.3.7 Intercalation and deintercalation
4.3.7.1 Graphite intercalation compounds
4.3.7.2 Pillared clays and layered double hydroxides
4.3.7.3 Synthesis of graphene
4.3.8 Example of a difficult synthesis made possible by chimie douce methods: BiFeO3
4.3.9 Molten salt synthesis, MSS
4.4 Gas‐Phase Methods
4.4.1 Vapour‐phase transport
4.4.2 Chemical vapour deposition, CVD
4.4.2.1 Amorphous silicon
4.4.2.2 Diamond films
4.4.3 Sputtering and evaporation, pulsed laser deposition, PLD and molecular beam epitaxy, MBE
4.4.4 Atomic layer deposition, ALD
4.4.5 Aerosol synthesis and spray pyrolysis
4.5 High‐Pressure Methods
4.6 Crystal Growth
4.6.1 Czochralski method
4.6.2 Bridgman and Stockbarger methods
4.6.3 Zone melting
4.6.4 Precipitation from solution or melt: flux method
4.6.5 Verneuil flame fusion method
4.6.6 Skull melting
5 Crystallography and Diffraction Techniques. 5.1 General Comments: Molecular and Non‐Molecular Solids. 5.1.1 Identification of crystalline solids
5.1.2 Structure of non‐molecular crystalline solids
5.1.3 Defects, impurities and stoichiometry of crystalline solids
5.2 Characterisation of Solids
5.3 X‐Ray Diffraction. 5.3.1 Generation of X‐rays. 5.3.1.1 Laboratory sources utilising inner shell electronic transitions
5.3.1.2 Synchrotron X‐ray sources
5.3.2 Interaction of X‐rays with matter
5.3.3 Optical grating and diffraction of light
5.3.4 Crystals and diffraction of X‐rays
5.3.4.1 The Laue equations
5.3.4.2 Bragg’s law
5.3.5 X‐ray diffraction methods
5.3.6 The powder method – principles and uses
5.3.6.1 Focusing of X‐rays: theorem of a circle
5.3.6.2 Crystal monochromators
5.3.6.3 Powder diffractometers
5.3.6.4 Guinier focusing cameras
5.3.6.5 A powder pattern of a crystalline phase is its ‘fingerprint’
5.3.6.6 Powder patterns and crystal structures
5.3.6.7 Powder patterns from single crystals: the Gandolfi technique
5.3.7 Intensities
5.3.7.1 Scattering of X‐rays by an atom: atomic scattering factors or form factors
5.3.7.2 Scattering of X‐rays by a crystal – systematic absences
5.3.7.3 General equation for phase difference, δ
5.3.7.4 Intensities and structure factors
5.3.7.5 Temperature factors
5.3.7.6 R‐factors and structure determination
5.3.7.7 Structure refinement from powder data: Rietveld refinement
5.3.8 X‐ray crystallography and structure determination ‐ what is involved?
5.3.8.1 The Patterson method
5.3.8.2 Fourier methods
5.3.8.3 Direct methods
5.3.8.4 Electron density maps
5.4 Electron Diffraction
5.5 Neutron Diffraction
5.5.1 Crystal structure determination
5.5.2 Magnetic structure analysis
5.5.3 Inelastic scattering, soft modes and phase transitions
5.6 The Reciprocal Lattice
5.6.1 Real and reciprocal lattices
5.6.2 Direct observation of the reciprocal lattice and systematic absences (extinctions)
5.6.3 Diffraction and the reciprocal lattice: the Ewald sphere of reflection
5.6.4 Selected area electron diffraction
5.7 Total scattering and pair distribution function (PDF) analysis
5.8 Line broadening of XRD powder patterns, domain (particle) size measurement and strain effects
6 Other Characterisation Techniques: Microscopy, Spectroscopy, Thermal Analysis
6.1 Diffraction and Microscopic Techniques: What Do They Have in Common?
6.2 Optical and Electron Microscopy Techniques. 6.2.1 Optical microscopy
6.2.1.1 Polarising microscope
6.2.1.2 Reflected light microscope
6.2.2 Electron microscopy
6.2.2.1 Scanning electron microscopy
6.2.2.2 Electron probe microanalysis, EPMA, and energy‐dispersive X‐ray spectroscopy, EDS or EDX
6.2.2.3 Auger electron (emission) microscopy and spectroscopy, AES
6.2.2.4 Cathodoluminescence, CL
6.2.2.5 Transmission electron microscopy, TEM, and scanning transmission electron microscopy, STEM
6.2.2.6 Electron energy loss spectroscopy, EELS
6.2.2.7 High‐angle annular dark field, HAADF/Z‐contrast STEM
6.3 Spectroscopic Techniques
6.3.1 Vibrational spectroscopy: IR and Raman
6.3.2 Visible and ultraviolet (UV) spectroscopy
6.3.3 Nuclear magnetic resonance (NMR) spectroscopy
6.3.4 Electron spin resonance (ESR) spectroscopy
6.3.5 X‐ray spectroscopies: XRF, AEFS, EXAFS
6.3.5.1 Emission techniques
6.3.5.2 Absorption techniques
XANES
EXAFS
6.3.6 Electron spectroscopies: ESCA, XPS, UPS, AES, EELS
6.3.7 Mössbauer spectroscopy
6.4 Thermal Analysis (TA)
6.4.1 Thermogravimetry (TG)
6.4.2 Differential thermal analysis (DTA) and differential scanning calorimetry (DSC)
6.4.3 Applications
6.5 Strategy to Identify, Analyse and Characterise ‘Unknown’ Solids
7 Phase Diagrams and Their Interpretation
7.1 The Phase Rule, the Condensed Phase Rule and Some Definitions
7.2 One‐Component Systems
7.2.1 The system H2O
7.2.2 The system SiO2
7.2.3 Condensed one‐component systems
7.3 Two‐Component Condensed Systems
7.3.1 A simple eutectic system
7.3.1.1 Liquidus and solidus
7.3.1.2 Eutectic
7.3.1.3 Lever rule
7.3.1.4 Eutectic reaction
7.3.1.5 The liquidus, saturation solubilities and freezing point depression
7.3.2 Binary systems with compounds
7.3.2.1 Congruent melting
7.3.2.2 Incongruent melting, peritectic point, peritectic reaction
7.3.2.3 Non‐equilibrium effects
7.3.2.4 Upper and lower limits of stability
7.3.3 Binary systems with solid solutions. 7.3.3.1 Complete solid solution
7.3.3.2 Fractional crystallisation
7.3.3.3 Thermal maxima and minima
7.3.3.4 Partial solid solution systems
7.3.4 Binary systems with solid–solid phase transitions
7.3.5 Binary systems with phase transitions and solid solutions: eutectoids and peritectoids
7.3.6 Binary systems with liquid immiscibility: MgO–SiO2
7.3.7 Some technologically important phase diagrams. 7.3.7.1 The system Fe–C: iron and steel making
7.3.7.2 The system CaO–SiO2: cement manufacture
7.3.7.3 The system Na–S: Na/S batteries
7.3.7.4 The system Na2O–SiO2: glass making
7.3.7.5 The system Li2O–SiO2: metastable phase separation and synthetic opals
7.3.7.6 Purification of semiconducting Si by zone refining
7.3.7.7 The system ZrO2–Y2O3: yttria‐stabilised zirconia, YSZ, solid electrolyte
7.3.7.8 The system Bi2O3–Fe2O3: multiferroic BiFeO3
7.4 Some Tips and Guidelines for Constructing Binary Phase Diagrams
7.5 Ternary Systems
7.5.1 Subsolidus equilibria
7.5.2 Subsolidus equilibria with solid solutions
7.5.3.1 Solid‐liquid phase diagrams. 7.5.3.1 Simple ternary eutectic system
7.5.3.2 Ternary systems containing binary compounds
7.5.3.3 Ternary systems containing solid solutions
7.5.3.3 Analogies between ternary phase diagrams, crystallisation pathways, geographical contour maps and hill walking
7.5.3.5 Some important ternary phase diagrams
7.6 Phase Transitions
7.6.1 What is a phase transition?
7.6.2 Buerger's classification: reconstructive and displacive transitions
7.6.3 Thermodynamic classification of phase transitions
7.6.4 Applications of G–T diagrams; stable and metastable phases
7.6.5 Representation of phase transitions on phase diagrams
7.6.6 Kinetics of phase transitions
7.6.7 Critical size of nuclei
7.6.8 Rate equations. 7.6.8.1 Nucleation rate
7.6.8.2 Overall transformation rate; the Avrami equation
7.6.8.3 Time–temperature–transformation (TTT) diagrams
7.6.9 Kinetics of phase transitions and solid state reactions
7.6.10 Crystal chemistry and phase transitions. 7.6.10.1 Structural changes with increasing temperature and pressure
7.6.10.2 Martensitic transformations
7.6.10.3 Order–disorder transitions
8 Electrical Properties. 8.1 Survey of Electrical Properties and Electrical Materials
8.2 Metallic Conductivity
8.2.1 Organic metals: conjugated systems. 8.2.1.1 Polyacetylene
8.2.1.2 Poly‐p‐phenylene and polypyrrole
8.2.2 Organic metals: charge‐transfer complexes
8.3 Superconductivity
8.3.1 The property of zero resistance
8.3.2 Perfect diamagnetism; the Meissner effect
8.3.3 Critical temperature Tc, critical field Hc and critical current Jc
8.3.4 Type I and type II superconductors: the vortex (mixed) state
8.3.5 Survey of superconducting materials
8.3.6 Crystal chemistry of cuprate perovskites
8.3.7 YBa2Cu3O7−δ, YBCO
8.3.7.1 Crystal structure
8.3.7.2 Atom valences and the superconducting mechanism
8.3.7.3 Oxygen content of YBa2Cu3O7−δ
8.3.7.4 Determination of oxygen content, 7−δ
8.3.8 Fullerides
8.3.9 Applications of superconductors
8.4 Semiconductivity
8.4.1 Elemental and compound semiconductors with diamond and zinc blende structures
8.4.2 Electrical properties of semiconductors
8.4.3 Oxide semiconductors
8.4.4 Applications of semiconductors
8.5 Ionic Conductivity
8.5.1 Alkali halides: vacancy conduction
8.5.1.1 Activation energy for ion hopping: geometric considerations
8.5.1.2 Ionic conductivity of NaCl crystals
8.5.1.3 Extrinsic conductivity in NaCl: control by aliovalent doping
8.5.2 Silver chloride: interstitial conduction
8.5.3 Alkaline earth fluorides
8.5.4 Solid electrolytes (or fast ion conductors, superionic conductors) 8.5.4.1 General considerations
8.5.4.2 β‐Alumina
Crystal structures of β‐ and β″‐aluminas
8.5.4.3 Nasicon
8.5.4.4 Hollandites and priderites
8.5.4.5 Silver and copper ion conductors
8.5.4.6 Fluoride ion conductors
8.5.4.7 Oxide ion conductors
8.5.4.8 Li+ ion conductors
8.5.4.9 Proton conductors
8.5.4.10 Mixed ionic/electronic conductors
8.5.4.11 Applications of solid electrolytes and mixed conductors
Thermodynamic measurements
Sodium‐sulphur and Zebra batteries
Miniature cells, heart pacemakers
Lithium batteries
Anodes
Electrolytes
Cathodes
Lithium‐air and lithium sulphur batteries
Electrochromic devices, smart windows
Gas sensors
Transport number measurements
Solid oxide fuel cells, SOFCs; steam electrolysers; oxygen pumps
8.6 Dielectric Materials
8.6.1 From dielectrics to conductors
8.7 Ferroelectrics
8.8 Pyroelectrics
8.9 Piezoelectrics
8.10 Applications of Ferro‐, Pyro‐ and Piezoelectrics
9 Magnetic Properties. 9.1 Physical Properties
9.1.1 Behaviour of substances in a magnetic field
9.1.2 Effects of temperature: Curie and Curie–Weiss laws
9.1.3 Magnetic moments
9.1.4 Mechanisms of ferro‐ and antiferromagnetic ordering: superexchange
9.1.5 Some more definitions
9.2 Magnetic Materials, their Structures and Properties. 9.2.1 Metals and alloys
9.2.2 Transition metal monoxides
9.2.3 Transition metal dioxides
9.2.4 Spinels
9.2.5 Garnets
9.2.6 Ilmenites and perovskites
9.2.7 Magnetoplumbites
9.3 Applications: Structure–Property Relations
9.3.1 Transformer cores
9.3.2 Permanent magnets
9.3.3 Magnetic information storage
9.4 Recent Developments. 9.4.1 Magnetoresistance: giant and colossal
9.4.2 Multiferroics
10 Optical Properties: Luminescence, Lasers and Transparent Conductors. 10.1 Visible Light and the Electromagnetic Spectrum
10.2 Sources of Light, Thermal Sources, Black Body Radiation and Electronic Transitions
10.3 Scattering Processes: Reflection, Diffraction and Interference
10.4 Luminescence and Phosphors
10.5 Configurational Coordinate Model
10.6 Some Phosphor Materials
10.7 Anti‐Stokes Phosphors
10.8 Stimulated Emission, Amplification of Light and Lasers
10.8.1 The ruby laser
10.8.2 Neodymium lasers
10.8.3 Semiconductor lasers and the light‐emitting diode, LED
10.9 Photodetectors
10.10 Fibre‐Optics
10.11 Solar Cells and Photovoltaics
10.12 Transparent Conducting Oxides, TCOs, Smart Windows and Energy Management of Buildings
10.12.1 Indium tin oxide, ITO
10.12.2 Applications
10.12.3 p‐type TCOs
10.13 Photonic Crystals, Photonic Bandgaps and Opals
11 Heterogeneous Materials, Electroceramics and Impedance Spectroscopy
11.1 Homogeneous and Heterogeneous Solids
11.2 Resistivities and Permittivities of Materials; The Parallel RC Element
11.3 Impedance Formalisms, Alternating Currents and Equivalent Circuits
11.4 Applications of Impedance Spectroscopy
12 Thermal and Thermoelectric Properties. 12.1 Thermoelectric Effects
12.1.1 Thermocouples
12.1.2 Thermoelectric power generation
12.2 Thermal Properties: Heat Capacity, Thermal Conductivity, Thermal Expansion. 12.2.1 Heat capacity
12.2.2 Thermal conductivity
12.2.3 Thermal expansion
13 Functional Materials: Some Important Examples
13.1 TiO2: Rutile, Anatase and Other Ti–O Phases
13.2 Ca12Al14O33, Mayenite: An Oxide Ion Conductor, Component of Cement and a Superconducting Electride
13.2.1 2D electrides
13.3 Zinc Oxide, ZnO for Varistor and Optoelectronic Applications
13.4 Two‐dimensional Structures: MXenes
13.5 Low‐dimensional Solids: Graphene, BN, Transition Metal Dichalcogenides and Black Phosphorus
14 Glass
14.1 Factors That Influence Glass Formation
14.1.1 Viscosity
14.1.2 Structural effects: Zachariasen’s rules
14.1.3 Other structural and bonding factors
14.2 Thermodynamics of Glass Formation; the Behaviour of Liquids on Cooling
14.3 Kinetics of Crystallisation and Glass Formation
14.4 Structure of Glasses
14.4.1 Vitreous silica and stochasticity
14.4.2 Silicate glasses
14.4.3 Vitreous B2O3 and borate glasses
14.5 Liquid Immiscibility and Phase Separation in Glasses
14.5.1 Structural theories of liquid immiscibility
14.5.2 Thermodynamics of liquid immiscibility
14.5.3 Mechanisms of phase separation: nucleation and growth; spinodal decomposition
14.6 Viscosity of Glasses and Melts
14.7 Electrical (Ionic) Conductivity of Glass and the Mixed Alkali Effect
14.8 Bonds, Bands and Semiconducting Glasses
14.8.1 Chalcogenide glasses
14.8.1.1 Sulphur
14.8.1.2 Selenium
14.8.1.3 Tellurium
14.8.1.4 More complex chalcogenide glasses: optical properties
14.8.2 Electrical properties
14.8.3 The photocopying process
14.8.4 Electrical switching and memory effects
14.9 Mechanical Properties and Strengthening of Glass
14.10 Commercial Silicate and Borate Glasses
14.10.1 Optical properties
14.11 Metallic Glasses
14.12 Fluoride Glasses
14.13 Glass‐Ceramics
14.13.1 Some important glass‐ceramic compositions
14.13.2 Properties of glass‐ceramics
14.13.3 Applications of glass‐ceramics
14.14 Bioglasses
15 Structural Materials: Cement, Refractories and Structural Ceramics. 15.1 Cements
15.1.1 Manufacture of Portland cement
15.1.2 Phase diagram considerations
15.1.3 Polymorphism and crystal structures of the calcium silicates and aluminates
15.1.4 Silicate chemistry and silicate structures
15.1.5 Hydration of Portland cement
15.1.6 Types of Portland cement
15.1.7 Alkali activation and geopolymers
15.1.8 Autoclaved products
15.1.9 Aluminous cement, high alumina cement and refractory concrete
15.1.10 Macro‐defect‐free (MDF) Cement
15.1.11 Acid‐base cements: oxychloride (Sorel) and glass‐ionomer cements
15.2 Refractories and Structural Ceramics
15.2.1 Microstructure or texture of refractories and ceramics
15.2.2 Refractory materials and structural ceramics
16 Oxides of the Elements, Their Properties and Uses
16.1 Oxides of s‐Block Elements
16.2 Acid‐Base Classification of Oxides
16.3 Oxides of p‐Block Elements
16.4 Oxides of d‐block (Transition) Elements
16.5 Oxides of Lanthanides and Actinides
16.6 Oxides of the Elements Overview. Actinium (Rn core) 7s26d1
Aluminium (Ne core) 3s23p1
Antimony (Kr core) 4d105s25p3
Arsenic (Ar core) 3d104s24p3
Barium (Xe core) 6s2
Beryllium (He core) 2s2
Bismuth (Xe core) 4f145d106s26p3
Boron (He core) 2s22p1
Bromine (Ar core) 3d104s24p5
Cadmium (Kr core) 4d105s2
Caesium (Xe core) 6s1
Calcium (Ar core) 4s2
Carbon (He core) 2s22p2
Cerium (Xe core) 4f15d16s2
Chlorine (Ne core) 3s23p5
Chromium (Ar core) 3d44s2
Cobalt (Ar core) 3d74s2
Copper (Ar core) 3d104s1
Dysprosium (Xe core) 4f106s2
Erbium (Xe core) 4f126s2
Europium (Xe core) 4f76s2
Gadolinium (Xe core) 4f75d16s2
Gallium (Ar core) 3d104s24p1
Germanium (Ar core) 3d104s24p2
Gold (Xe core) 4f145d106s1
Hafnium (Xe core) 4f145d26s2
Holmium (Xe core) 4f116s2
Indium (Kr core) 3d104s24p1
Iodine (Kr core) 4d105s25p5
Iridium (Xe core) 4f145d76s2
Iron (Ar core) 3d64s2
Lanthanum (Xe core) 5d16s2
Lead (Xe core) 4f145d106s26p2
Lithium (He core) 2s1
Lutetium (Xe core) 4f145d16s2
Magnesium (Ne core) 3s2
Manganese (Ar core) 3d54s2
Mercury (Xe core) 4f145d106s2
Molybdenum (Kr core) 4d55s1
Neodymium (Xe core) 4f46s2
Nickel (Ar core) 3d84s2
Niobium (Kr core) 4d35s2
Nitrogen (He core) 2s22p3
Osmium (Xe core) 4f145d66s2
Palladium (Kr core) 4d10
Phosphorus (Ne core) 3s23p3
Platinum (Xe core) 4f145d96s1
Polonium (Xe core) 4f145d106s26p4
Potassium (Ar core) 4s1
Praseodymium (Xe core) 4f36s2
Promethium (Xe core) 4f56s2
Protactinium (Rn core) 5f26d17s2
Rhenium (Xe core) 4f145d56s2
Rhodium (Kr core) 4d85s1
Rubidium (Kr core) 5s1
Ruthenium (Kr core) 4d75s1
Samarium (Xe core) 4f66s2
Scandium (Ar core) 3d14s2
Selenium (Ar core) 3d104s24p4
Silicon (Ne core) 3s23p2
Silver (Kr core) 5s14d10
Sodium (Ne core) 3 s 1
Strontium (Kr core) 5s2
Sulphur (Neon core) 3s23p4
Tantalum (Xe core) 4f145d36s2
Technetium (Kr core) 4d55s2
Tellurium (Kr core) 4d105s25p4
Terbium (Xe core) 4f96s2
Thallium (Xe core) 4f145d106s26p1
Thorium (Rn core) 6d27s2
Thulium (Xe core) 4f136s2
Tin (Kr core) 3d104s24p2
Titanium (Ar core) 3d24s2
Tungsten (Xe core) 4f145d46s2
Uranium (Rn core) 5f36d17s2
Vanadium (Ar core) 3d34s2
Xenon (Kr core) 4d105s25p6
Ytterbium (Xe core) 4f146s2
Yttrium (Kr core) 4d15s2
Zinc (Ar core) 3d104s2
Zirconium (Kr core) 4d25s2
Appendix A Interplanar Spacings and Unit Cell Volumes
Appendix B Model Building
Equipment Needed
Sphere Packing Arrangements
To show the relation between a ccp structure and an fcc unit cell
To show the cp layers and unit cell of an hcp stacking sequence
Polyhedral structures
Appendix C Geometrical Considerations in Crystal Chemistry. Notes on the Geometry of Tetrahedra and Octahedra. Relation of a tetrahedron to a cube
Relation between distances M–X and X–X in a tetrahedron
Angle XMX of a tetrahedron
Symmetry of a tetrahedron
Centre of gravity of a tetrahedron
Relation of an octahedron to a cube
Hexagonal unit cell: proof that the axial ratio, c/a, ideally equals 1.633
Appendix D The Elements and Some of Their Properties
Appendix E The 32 Crystallographic Point Groups
Appendix F The Arrhenius Equation for Ionic Conductivity
Reference
Appendix G A Guide to the Use of Electrode Potentials
Further Reading
General
Chapter 1
Chapter 2
Chapter 3
Chapter 4
Chapter 5
Chapter 6
Chapter 7
Chapter 8
Chapter 9
Chapter 10
Chapter 11
Chapter 12
Chapter 13
Chapter 14
Chapter 15
Chapter 16
Questions. Questions
Index
WILEY END USER LICENSE AGREEMENT
Отрывок из книги
Second Edition
ANTHONY R. WEST
.....
The need to characterise solid state materials across the length scales from atomic through to macro is, however, part of a much bigger picture that includes the targeted synthesis of materials with different dimensionality and size. This takes us into the realms of nanomaterials and nanotechnology. Nanomaterials have reduced particle size and much increased net surface area, but also include many examples of low dimensionality, typified by the carbon nanostructures of zero‐dimensional fullerenes C60 and C70, one‐dimensional carbon nanotubes and two‐dimensional graphene sheets.
Physical properties of nanomaterials often diverge from those expected based on the known behaviour of macro‐sized and three‐dimensional materials. Thus, quantum confinement effects lead to band structure modification and variable electronic and optical properties in colloidal systems. The importance of surface structure and properties is increasingly dominant in nano‐sized materials because most of the material is close to, or at, a surface. Surface structures always differ from bulk structures because of modified coordination environments and bonding arrangements, but in the study of bulk materials, surface effects are frequently ignored in favour of the dominant bulk structure and properties. They cannot be ignored in nanomaterials however, because nano‐size local bonding/structure effects underpin industries that are often based on and include heterogeneous catalysis, sensors, smart windows and solar cell technology.
.....