Structure and Function of the Bacterial Genome
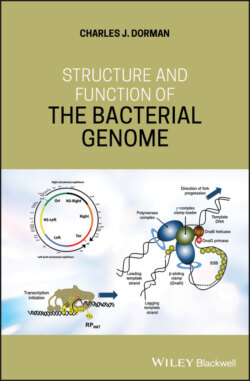
Реклама. ООО «ЛитРес», ИНН: 7719571260.
Оглавление
Charles J. Dorman. Structure and Function of the Bacterial Genome
Table of Contents
List of Tables
List of Illustrations
Guide
Pages
Structure and Function of the Bacterial Genome
Copyright
Preface
1 The Bacterial Genome – Where the Genes Are
1.1 Genome Philosophy
1.2 The Bacterial Chromosome
1.3 Chromosome Replication: Initiation
1.4 Chromosome Replication: Elongation
1.5 Chromosome Replication: Termination
1.6 Replication Produces Physically Connected Products
1.7 Decatenating the Sister Chromosomes
1.8 Resolving Chromosome Dimers
1.9 Segregating the Products of Chromosome Replication
1.10 Polar Tethering of Chromosome Origins
1.11 Some Bacterial Chromosomes Are Linear
1.12 Some Bacteria Have More than One Chromosome
1.13 Plasmids
1.14 Plasmid Replication
1.15 Plasmid Segregation
1.16 The Nucleoid
1.17 The Chromosome Has Looped Domains
1.18 The Macrodomain Structure of the Chromosome
1.19 The Chromosome Displays Spatial Arrangement Within the Cell
1.20 SeqA and Nucleoid Organisation
1.21 MukB, a Condensin‐Like Protein
1.22 MatP, the matS Site and Ter Organisation
1.23 MaoP and the maoS Site
1.24 SlmA and Nucleoid Occlusion
1.25 The Min System and Z Ring Localisation
1.26 DNA in the Bacterial Nucleoid
1.27 DNA Topology
1.28 DNA Topoisomerases: DNA Gyrase
1.29 DNA Topoisomerases: DNA Topoisomerase IV
1.30 DNA Topoisomerases: DNA Topoisomerase I
1.31 DNA Topoisomerases: DNA Topoisomerase III
1.32 DNA Replication and Transcription Alter Local DNA Topology
1.33 Transcription and Nucleoid Structure
1.34 Nucleoid‐associated Proteins (NAPs) and Nucleoid Structure
1.35 DNA Bending Protein Integration Host Factor (IHF)
1.36 HU, a NAP with General DNA‐binding Activity
1.37 The Very Versatile FIS Protein
1.38 FIS and the Early Exponential Phase of Growth
1.39 FIS and the Stringent Response
1.40 FIS and DNA Topology
1.41 Ferritin‐Like Dps and the Curved‐DNA‐binding Protein CbpA
1.42 The H‐NS Protein: A Silencer of Transcription
1.43 StpA: A Paralogue of H‐NS
1.44 H‐NS Orthologues Encoded by Plasmids and Phage
1.45 H‐NSB/Hfp and H‐NS2: H‐NS Homologues of HGT Origin
1.46 A Truncated H‐NS‐Like Protein
1.47 Hha‐like Proteins
1.48 Other H‐NS Homologues: The Ler Protein from EPEC
1.49 H‐NS Functional Homologues
1.50 H‐NS Functional Homologues: Rok from Bacillus spp
1.51 H‐NS Functional Homologues: Lsr2 from Actinomycetes
1.52 H‐NS Functional Homologues: MvaT from Pseudomonas spp
1.53 The Leucine‐responsive Regulatory Protein, LRP
1.54 Small, Acid‐soluble Spore Proteins, SASPs
2 Conservation and Evolution of the Dynamic Genome
2.1 Disruptive Influences: Mutations
2.2 Repetitive Sequences in the Chromosome and Their Influence on Genetic Stability
2.3 Contingency Loci and the Generation of Microbial Variety
2.4 Rhs: Rearrangement Hotspots
2.5 REP Sequences
2.6 RIB/RIP, BIME‐1, and BIME‐2 Elements
2.7 ERIC Sequences
2.8 Repeat‐Mediated Rearrangements: Mechanisms and Frequency
2.9 Site‐specific Recombination and Phenotypic Variety
2.10 Site‐Specific Recombination: Bacteriophage Lambda
2.11 The Lambda Lysis/Lysogeny Decision
2.12 Tyrosine Integrases
2.13 Serine Invertases
2.14 Large Serine Recombinases
2.15 Transposition and Transposable Elements
2.16 Pathways of Transposition
2.17 Peel‐and‐paste Transposition
2.18 Control of Transposition
2.19 Host Factors and Transposition
2.20 Integrative and Conjugative Elements (ICE)
2.21 Integrons
2.22 Introns
2.23 Horizontal Gene Transfer
2.24 Distinguishing Self from Non‐self
2.25 Distinguishing Self and Non‐self: CRISPR‐Cas Systems
2.26 Distinguishing Self and Non‐self: Argonaute Proteins
2.27 Distinguishing Self and Non‐self: Restriction Enzymes/Methylases
2.28 Distinguishing Self and Non‐self: BREX
2.29 Self‐sacrifice and Other Behaviours Involving Toxin—antitoxin Systems
2.30 Conservative Forces: DNA Repair and Homologous Recombination
2.31 The RecA Protein
2.32 RecA, LexA, and the SOS Response
2.33 Holliday Junction Resolution
2.34 Mismatch Repair
2.35 Non‐homologous End Joining
3 Gene Control: Transcription and Its Regulation
3.1 Transcription: More Than Just Transcribing Genetic Information
3.2 RNA Polymerase
3.3 The Core Enzyme
3.4 The Sigma Factors (and Anti‐Sigma Factors)
3.5 Promoter Architecture
3.6 Stringently Regulated Promoters
3.7 Transcription Factors and RNA Polymerase
3.8 Transcription Initiation
3.9 Transcription Elongation
3.10 Transcription Termination: Intrinsic and Rho‐Dependent Terminators
3.11 Rho and Imported Genes
3.12 Rho, R‐Loops, and DNA Supercoiling
3.13 Rho and Antisense Transcripts
3.14 Anti‐Termination: Insights from Phage Studies
3.15 Transcription Occurs in Bursts
4 Gene Control: Regulation at the RNA Level
4.1 Antisense Transcripts and Gene Regulation incis
4.2 RNA that Regulates intrans
4.3 DsrA and the RpoS/H‐NS Link
4.4 sRNA Turnover
4.5 DEAD‐box Proteins
4.6 RNA Chaperone Proteins
4.7 StpA, H‐NS, and RNA Binding
4.8 Degradation of mRNA
4.9 RNA Folding and Gene Regulation
4.10 Transcription Attenuation
4.11 Riboswitches
4.12 RNA as a Structural Component in the Nucleoid
5 Gene Control: Regulation at the Protein Level. 5.1 Control Beyond DNA and RNA
5.2 Translation Machinery and Control: tRNA and rRNA
5.3 Translation Machinery and Control: The Ribosome
5.4 Translation Initiation
5.5 Translation Elongation
5.6 Elongation Factor P (EF‐P)
5.7 Translation Termination
5.8 Protein Secretion
5.9 Protein Secretion: The Sec Pathway
5.10 The Twin Arginine Translocation (Tat) Pathway of Protein Secretion
5.11 Type 1 Secretion Systems (T1SS)
5.12 Type 2 Secretion Systems (T2SS)
5.13 Type 3 Secretion Systems (T3SS)
5.14 Type 4 Secretion Systems (T4SS)
5.15 Type 5 Secretion Systems (T5SS): The Autotransporters
5.16 Type 6 Secretion Systems (T6SS)
5.17 Protein Secretion in Gram‐Positive Bacteria: SecA1, SecA2, and SrtA
5.18 Type 7 Secretion Systems (T7SS)
5.19 Protein Modification: Acetylation
5.20 Protein Modification: Glycosylation
5.21 Protein Modification: Phosphorylation
5.22 Protein Splicing
5.23 Small Proteins
5.24 Selenocysteine and Pyrrolysine: The 21st and 22nd Amino Acids
6 Gene Control and Bacterial Physiology. 6.1 The Bacterial Growth Cycle
6.2 Physiology Changes Throughout the Growth Cycle
6.3 Generating Physiological Variety from Genetic Homogeneity
6.4 Bacterial Economics – Some Basic Principles
6.5 Carbon Sources and Metabolism
6.6 Gene Control and Carbon Source Utilisation
6.7 Anaerobic Respiration
6.8 ArcA, Mobile Genetic Elements, and HGT
6.9 Stress and Stress Survival in Bacterial Life
6.10 Oxygen Stress
6.11 Iron Starvation
6.12 Siderophores and Iron Capture
6.13 TonB‐Dependent Transporters
6.14 Gene Regulation and Iron Transport
6.15 Iron Storage and Homeostasis
6.16 Osmotic Stress and Water Relations in Bacteria
6.17 Signal Molecules and Stress
6.18 The Stringent Response
6.19 Regulation of the Acid Stress Response
6.20 Alkaline pH Stress Response
6.21 Motility and Chemotaxis
6.22 Quorum Sensing
6.23 Biofilms
6.24 ‘Cheating’ as a Lifestyle Strategy
6.25 Thermal Regulation
6.26 Epigenomics and Phasevarions
6.27 Some Unifying Themes
7 Gene Control: Global Regulation by H‐NS. 7.1 H‐NS Is a Global Regulator
7.2 H‐NS and Foreign DNA
7.3 H‐NS and Xenogenic Silencing: Three Case Studies
7.4 The H‐NS Virulence Regulon in Vibrio cholerae
7.5 HGT in V. cholerae: The CTXφ Phage and the VPI1 Island
7.6 The ToxRS, ToxT, TcpPH Regulatory Network
7.7 Control by VpsR, VpsT, and HapR
7.8 Quorum Sensing and Cholera
7.9 Chitin and HGT
7.10 The H‐NS Virulence Regulon in Shigella flexneri
7.11 Shigella Infection
7.12 The VirF AraC‐Like Transcription Factor
7.13 VirB: A Recruit from a Plasmid‐Partitioning System
7.14 The Shigella Virulence Plasmid
7.15 The Salmonella H‐NS Virulence Gene Regulon
7.16 Salmonella's Pathogenicity Islands (SPI)
7.17 SlyA, PhoP/Q, and SPI Gene Expression
7.18 Gene Control in SPI1 and SPI2
8 An Integrated View of Genome Structure and Function. 8.1 Networks versus Hierarchies
8.2 Regulons, Stimulons, and Heterarchies/Netarchies
8.3 Transcription Burstiness and Regulatory Noise
8.4 The Significance of Gene Position
8.5 Messenger RNA May Not Be Free to Diffuse Far in Bacteria
8.6 RNA Polymerase Activity and Genome Organisation
8.7 Gene–Gene Interactions in the Folded Chromosome
8.8 DNA Supercoiling as a Global Regulator
8.9 Modelling the Nucleoid
8.10 Synthetic Biology
References
Index
WILEY END USER LICENSE AGREEMENT
Отрывок из книги
Charles J. Dorman
Department of Microbiology,
.....
MukB forms topologically stable loops in the chromosomal DNA and protects the supercoils in these protected loops (Kumar et al. 2017a). It forms a complex with the MukE and MukF proteins, with these seeming to play a role in the turnover of the MukB complex on DNA in combination with the ATPase activity of MukB itself (Kumar et al. 2017a). MukF performs a bridging role between the ATPase heads of the two MukB in the complex (Figure 1.10). Proteins performing this task in SMC complexes are called kleisins. The equivalent system in B. subtilis and C. crescentus consists of the proteins Smc (MukB), ScpA (MukF, kleisin), and ScpB (MukE): the ‘Scp’ designation indicates that the protein is a ‘segregation and condensation protein’ (Britton et al. 1998; Burmann et al. 2013; Jensen and Shapiro 1999, 2003; Mascarenhas et al. 2005).
The MukBEF complex has important architectural and segregational roles in the nucleoid, operating mainly outside the Ter macrodomain of the chromosome. Its principal site of action seems to be at ori and MukB requires MukE, MukF, and ATP hydrolysis to gain and maintain this association; MukBEF/SMC complexes do not seem to track moving replisomes (Badrinarayanan et al. 2012a,b; Danilova et al. 2007; Gruber and Errington 2009; Sullivan et al. 2009). MukBEF is responsible for guiding the newly replicated ori regions into the two halves of the cell, driving bipolar segregation; if MukBEF is removed, the ori shifts from mid‐cell to the pole, disturbing normal chromosome orientation and segregation patterns (Danilova et al. 2007). Inside the Ter macrodomain, the MatP protein prevents MukBEF from playing a structural role by displacing it and so making it available for ori binding (Lioy et al. 2018; Nolivos and Sherratt 2014; Nolivos et al. 2016). The MukBEF complex is not required for sister chromosome cohesion because muk mutants have a higher degree of cohesion of sister chromosomes than wild‐type cells (Danilova et al. 2007).
.....