Antenna-in-Package Technology and Applications
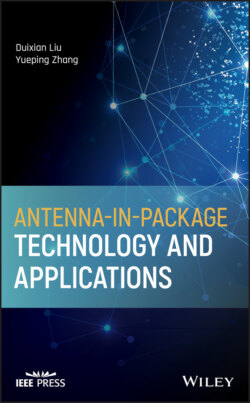
Реклама. ООО «ЛитРес», ИНН: 7719571260.
Оглавление
Duixian Liu. Antenna-in-Package Technology and Applications
Table of Contents
List of Tables
List of Illustrations
Guide
Pages
Antenna-in-Package Technology and Applications
List of Contributors
Preface
Abbreviations
Symbols
1 Introduction
1.1 Background
1.2 The Idea
1.3 Exploring the Idea
1.3.1 Bluetooth Radio and Other RF Applications
1.3.2 60‐GHz Radio and Other Millimeter‐wave Applications
1.4 Developing the Idea into a Mainstream Technology
1.5 Concluding Remarks
Acknowledgements
References
2 Antennas
2.1 Introduction
2.2 Basic Antennas. 2.2.1 Dipole
2.2.2 Monopole
2.2.3 Loop
2.2.4 Slot
2.3 Unusual Antennas. 2.3.1 Laminated Resonator Antenna
2.3.2 Dish‐like Reflector Antenna
2.3.3 Slab Waveguide Antenna
2.3.4 Differentially Fed Aperture Antenna
2.3.5 Step‐profiled Corrugated Horn Antenna
2.4 Microstrip Patch Antennas
2.4.1 Basic Patch Antennas
2.4.2 Stacked Patch Antennas
2.4.3 Patch Antenna Arrays
2.5 Microstrip Grid Array Antennas
2.5.1 Basic Configuration
2.5.2 Principle of Operation
2.5.3 Design Formulas with an Example
2.6 Yagi‐Uda Antennas
2.6.1 Horizontal Yagi‐Uda Antenna
2.6.2 Vertical Yagi‐Uda Antenna
2.6.3 Yagi‐Uda Antenna Array
2.7 Magneto‐Electric Dipole Antennas
2.7.1 Single‐polarized Microstrip Magneto‐electric Dipole Antenna
2.7.2 Dual‐polarized Microstrip Magneto‐electric Dipole Antenna
2.7.3 Simulated and Measured Results
2.8 Performance Improvement Techniques
2.8.1 Single‐layer Spiral AMC
2.8.2 Design Guidelines
2.8.3 A Design Example
2.9 Summary
Acknowledgements
References
3 Packaging Technologies
3.1 Introduction
3.2 Major Packaging Milestones
3.3 Packaging Taxonomy
3.3.1 Routing Layer in Packages
3.3.1.1 Lead Frame
3.3.1.2 Laminate
3.3.1.3 Redistribution Layer
3.3.2 Die to Routing Layer Interconnect
3.3.2.1 Wire Bonds
3.3.2.2 Flip Chips
3.4 Packaging Process for Several Major Packages
3.4.1 Wire Bond Plastic Ball Grid Array
3.4.1.1 Die Preparation
3.4.1.2 Die Attach
3.4.1.3 Wire Bonding
3.4.1.4 Molding
3.4.1.5 Ball Mounting
3.4.1.6 Package Singulation
3.4.2 Wire Bond Quad Flat No‐Lead Packages
3.4.3 Flip‐chip Plastic Ball Grid Arrays
3.4.3.1 Flip‐chip Bumping
3.4.3.1.1 Solder Bumping
3.4.3.1.2 Cu Pillar Bumping
3.4.3.2 Flip‐chip Attach
3.4.3.3 Underfill
3.4.4 Wafer Level Packaging
3.4.5 Fan Out Wafer Level Packaging
3.5 Summary and Emerging Trends
References
4 Electrical, Mechanical, and Thermal Co‐Design
4.1 Introduction
4.2 Electrical, Warpage, and Thermomechanical Analysis for AiP Co‐design. 4.2.1 28‐GHz Phased Array Antenna Module Overview
4.2.2 Thermomechanical Test Vehicle Overview
4.2.3 Antenna Prototyping and Interconnect Characterization
4.2.4 Warpage Analysis and Test
4.2.5 Thermal Simulation and Characterization
4.3 Thermal Management Considerations for Next‐generation Heterogeneous Integrated Systems. 4.3.1 AiP Cooling Options Under Different Power Dissipation Conditions
4.3.2 Thermal Management for Heterogeneous Integrated High‐power Systems
Acknowledgment
References
5 Antenna‐in‐Package Measurements
5.1 General Introduction and Antenna Parameters. 5.1.1 Antenna Measurement Concepts
5.1.2 Field Regions
5.1.3 Radiation Characteristics
5.1.4 Polarization Properties of Antennas
5.2 Impedance Measurements. 5.2.1 Circuit Representation of Antennas
5.3 Anechoic Measurement Facility for Characterizing AiPs
5.3.1 Design of the mmWave Anechoic Chamber
5.3.2 Defining Antenna Measurement Uncertainty
5.3.3 Uncertainty in the mmWave Antenna Test Facility
5.3.4 Case Study AiP: Characterization of a mmWave Circularly Polarized Rod Antenna
5.4 Over‐the‐air System‐level Testing
5.5 Summary and Conclusions
References
Note
6 Antenna‐in‐package Designs in Multilayered Low‐temperature Co‐fired Ceramic Platforms
6.1 Introduction
6.2 LTCC Technology
6.2.1 Introduction
6.2.2 LTCC Fabrication Process
6.2.3 LTCC Material Suppliers and Manufacturing Foundries
6.3 LTCC‐based AiP
6.3.1 SIW AiP
6.3.2 mmWave AiP
6.3.2.1 5G AiP
6.3.2.2 WPAN (60‐GHz) AiP
6.3.2.3 Automotive Radar (79‐GHz) AiP
6.3.2.4 Imaging and Radar (94‐GHz) AiP
6.3.2.5 Sub‐THz (Above‐100‐GHz) AiP
6.3.3 Active Antenna in LTCC
6.3.4 Gain Enhancement Techniques in LTCC
6.3.5 Ferrite LTCC‐based Antenna
6.4 Challenges and Upcoming Trends in LTCC AiP
References
7 Antenna Integration in Packaging Technology operating from 60 GHz up to 300 GHz (HDI‐based AiP)
7.1 Organic Packaging Technology for AiP
7.1.1 Organic Package Overview
7.1.2 Buildup Architecture
7.1.3 Industrial Material
7.1.4 HDI Design Rules
7.1.5 Assembly Constraints and Body Size
7.2 Integration of AiP in Organic Packaging Technology Below 100 GHz. 7.2.1 Integration Strategy of the Antenna
7.2.2 60‐GHz AiP Modules
7.2.3 94‐GHz AiP Module
7.3 Integration of AiP in Organic Packaging Technology in the 120–140‐GHz Band
7.3.1 120–140‐GHz AiP Module
7.3.2 Link Demonstration Using a BiCMOS Chip with the 120‐GHz BGA Module
7.4 Integration of AiP in Organic Packaging Technology Beyond 200 GHz
7.5 Conclusion and Perspectives
References
8 Antenna Integration in eWLB Package
8.1 Introduction
8.2 The Embedded Wafer Level BGA Package
8.2.1 Process Flow for the eWLB
8.2.2 Vertical Interconnections in the eWLB
8.2.3 Embedded Z‐Line Technology
8.3 Toolbox Elements for AiP in eWLB
8.3.1 Transmission Lines
8.3.2 Passive Components and Distributed RF Circuits
8.3.3 RF Transition to PCB
8.3.4 Vertical RF Transitions
8.4 Antenna Integration in eWLB
8.4.1 Single Antenna
8.4.2 Antenna Array
8.4.3 3D Antenna and Antenna Arrays
8.5 Application Examples
8.5.1 Two‐channel 60‐GHz Transceiver Module
8.5.2 Four‐channel 77‐GHz Transceiver Module
8.5.3 Six‐channel 60‐GHz Transceiver Module
8.6 Conclusion
Acknowledgement
References
NOTE
9 Additive Manufacturing AiP Designs and Applications
9.1 Introduction
9.2 Additive Manufacturing Technologies. 9.2.1 Inkjet Printing
9.2.2 FDM 3D Printing
9.2.3 SLA 3D Printing
9.3 Material Characterization
9.3.1 Resonator‐based Material Characterization
9.3.2 Transmissive‐based Material Characterization
9.4 Recent Advances in AM for Packaging
9.4.1 Interconnects
9.4.2 AiP
9.5 Fabrication Process. 9.5.1 3D Printing Process
9.5.2 Inkjet Printing Process
9.5.3 AiP Fabrication Process
9.6 AiP and SoP using AM Technologies. 9.6.1 AiP Design
9.6.2 SoP Design
9.7 Summary and Prospect
References
10 SLC‐based AiP for Phased Array Applications
10.1 Introduction
10.2 SLC Technology
10.3 AiP for 5G Base Station Applications
10.3.1 Package and Antenna Structure
10.3.2 AiP Design Considerations. 10.3.2.1 Surface Wave Effects
10.3.2.2 Vertical Transitions
10.3.3 Aperture‐coupled Patch Antenna Design
10.3.4 28‐GHz Aperture‐coupled Cavity‐backed Patch Array Design
10.3.5 Passive Antenna Element Characterization
10.3.6 Active Module Characterization of 64‐element Beams
10.3.7 28‐GHz AiP Phased‐array Conclusion
10.4 94‐GHz Scalable AiP Phased‐array Applications
10.4.1 Scalable Phased‐array Concept
10.4.2 94‐GHz Antenna Prototype Designs
10.4.3 94‐GHz Antenna Prototype Evaluation
10.4.4 94‐GHz AiP Array Design
10.4.5 Package Modeling and Simulation
10.4.6 Package Assembly and Test
10.4.7 Antenna Pattern and Radiated Power Measurement
Acknowledgment
References
11 3D AiP for Power Transfer, Sensor Nodes, and IoT Applications
11.1 Introduction
11.2 Small Antenna Design and Miniaturization Techniques
11.2.1 Physical Bounds on the Radiation Q‐factor for Antenna Structures
11.2.1.1 Lower Bounds on Antenna Enclosed in a Sphere: Chu, McLean, and Thal Limits
11.2.1.2 Lower Bounds on Antenna Enclosed in an Arbitrary Structure: Gustafsson–Yaghjian Limit
11.2.2 Figure of Merit for Antenna Miniaturization. 11.2.2.1 Relation between Q‐factor and Antenna Input Impedance
11.2.2.2 Antenna Efficiency Effect on the Radiation Q
11.2.2.3 Cross‐polarization Effect on Antenna Radiation Q
11.2.2.4 Figure of Merit Definition
11.2.3 Antenna Miniaturization Techniques
11.2.3.1 Miniaturization through Geometrical Shaping of the Antenna
11.2.3.1.1 Slot Loading
11.2.3.1.2 Pin or Plate Loading
11.2.3.1.3 Inductive Loading
11.2.3.1.4 Capacitive Loading
11.2.3.2 Miniaturization through Material Loading
11.2.3.2.1 Loading through Dielectric or Ferromagnetic Materials
11.2.3.2.2 Loading through Metamaterials
11.2.3.2.3 Loading through Lumped‐Element RLC Circuits
11.2.3.2.4 Miniaturization by Slow‐wave Meanderline Loading
11.3 Multi‐mode Capability: A Way to Achieve Wideband Antennas
11.4 Miniaturized Antenna Solutions for Power Transfer and Energy Harvesting Applications
11.4.1 Integrated Antenna Design Challenges for WPT and Scavenging Systems. 11.4.1.1 Conjugate Impedance Matching
11.4.1.2 Antenna Structure Selection
11.4.2 Small Antenna Structure that can be Optimized for Arbitrary Input Impedance. 11.4.2.1 Basic Antenna Structure
11.4.2.2 Antenna Size Reduction by Folding
11.4.2.3 Final Antenna Structure and Parameter Analysis
11.4.3 Example of an AiP Solution for On‐chip Scavenging/UWB Applications
11.5 AiP Solutions in Low‐cost PCB Technology
11.5.1 Introduction to Wireless Sensor Networks and IoT
11.5.1.1 Examples of Antennas for IoT Devices
11.5.2 3D System‐in‐Package Solutions for Microwave Wireless Devices
11.5.3 E‐CUBE: A 3D SiP Solution
11.5.3.1 Multilayer Flex‐rigid PCB for Antenna Element Design
11.5.3.2 Modular Design of the Antenna Array and Power Distribution Network
11.5.3.3 Construction and Measurement Results
References
Index
WILEY END USER LICENSE AGREEMENT
Отрывок из книги
IEEE Press
.....
Technology R&D
Silicon Technology Development
.....