Metal Additive Manufacturing
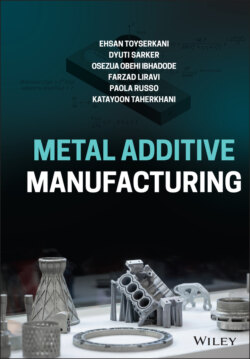
Реклама. ООО «ЛитРес», ИНН: 7719571260.
Оглавление
Ehsan Toyserkani. Metal Additive Manufacturing
Table of Contents
List of Tables
List of Illustrations
Guide
Pages
METAL ADDITIVE MANUFACTURING
Preface
Abbreviations
Nomenclature
Greek Symbols
1 Additive Manufacturing Process Classification, Applications, Trends, Opportunities, and Challenges. Learning Objectives
1.1 Additive Manufacturing: A Long‐Term Game Changer
1.2 AM Standard Definition and Classification
1.3 Why Metal Additive Manufacturing?
1.4 Market Size: Current and Future Estimation
1.5 Applications of Metal AM
1.5.1 Medical and Dental
1.5.2 Aerospace and Defense
1.5.3 Communication
1.5.4 Energy and Resources
1.5.5 Automotive
1.5.6 Industrial Tooling and Other Applications
1.6 Economic/Environmental Benefits and Societal Impact
1.7 AM Trends, Challenges, and Opportunities
1.8 Looking Ahead
References
2 Basics of Metal Additive Manufacturing. Learning Objectives
2.1 Introduction
2.2 Main Metal Additive Manufacturing Processes
2.2.1 Powder Bed Fusion (PBF)
2.2.1.1 PBF Process Description
2.2.1.2 PBF Advantages and Challenges
2.2.1.3 Commercial PBF Systems
2.2.2 Directed Energy Deposition (DED) 2.2.2.1 DED Process Description
2.2.2.2 DED Advantages and Challenges
2.2.2.3 Commercial DED Systems
2.2.3 Binder Jetting (BJ) 2.2.3.1 BJ Process Description
2.2.3.2 BJ Advantages and Challenges
2.2.3.3 Commercial BJ Systems
2.2.4 Emerging Metal AM Processes
2.2.4.1 Material Extrusion
2.2.4.2 Material Jetting
2.2.4.3 Sheet Lamination
2.3 Main Process Parameters for Metal DED, PBF, and BJ
2.3.1 Main Output Parameters
2.3.1.1 Melt Pool Dimensional Characteristics
2.3.1.2 Dilution
2.3.2 Combined Thermal Energy Source Parameters for PBF and DED
2.3.3 Beam Scanning Strategies and Parameters for PBF and DED
2.3.4 Powder Properties for PBF, DED, and BJ
2.3.4.1 Methods of Powder Particles Production
2.3.5 Wire Properties for DED
2.3.6 Layer Thickness for PBF, DED, and BJ
2.3.7 Ambient Parameters for PBF, DED, and BJ
2.3.8 Geometry‐Specific Parameters (PBF)
2.3.9 Support Structures for PBF
2.3.10 Binder Properties for BJ
2.3.11 Binder Saturation for BJ
2.4 Materials
2.4.1 Ferrous Alloys
2.4.2 Titanium Alloys
2.4.3 Nickel Alloys
2.4.4 Aluminum Alloys
References
3 Main Sub‐Systems for Metal AM Machines. Learning Objectives
3.1 Introduction
3.2 System Setup of AM Machines. 3.2.1 Laser Powder Bed Fusion (LPBF)
3.2.2 Laser Directed Energy Deposition (LDED) with Blown Powder Known as Laser Powder‐Fed (LPF)
3.2.3 Binder Jetting (BJ)
3.3 Laser Basics: Important Parameters Needed to be Known for AM
3.3.1 Laser Theory
3.3.1.1 Rate Equations and Einstein Coefficients
3.3.1.2 The Two‐Level System
3.3.1.3 The Three‐Level System
3.3.1.4 The Four‐Level System
3.3.2 Laser Components
3.3.2.1 Gain Medium
3.3.2.2 Pumping Source
3.3.2.3 Resonant Optical Cavity
3.3.3 Continuous Vs. Pulsed Laser
3.3.4 Laser Types
3.3.4.1 Solid‐State Lasers
Nd:YAG Solid‐State Laser
Disk Lasers
3.3.4.2 Gas Lasers
CO2 Laser
3.3.4.3 Liquid Dye Lasers
3.3.4.4 Semiconductor Diode Lasers
3.3.4.5 Fiber Optic Lasers
Yb‐ and Er‐Fiber Laser
3.3.4.6 Laser Deployed in Laser‐Based AM Processes
3.3.5 Laser Beam Properties
3.4 Electron Beam Basics
3.4.1 Comparisons and Contrasts between Laser and Electron Beams
3.4.2 Electron Beam Powder Bed Fusion Setup
3.4.3 Electron Beam Mechanism
3.4.3.1 Electron Beam Sources
3.4.3.2 Electron Beam Optics and Positioning
Electromagnetic Lens
Stigmators
Rasterizer
3.4.4 Vacuum Chambers
3.4.4.1 Vacuum Types and Pumping Systems for EBM
3.4.4.2 Chamber Types for EBM
3.5 Powder Feeders and Delivery Nozzles Technology
3.5.1 Classification of Powder Feeders
3.5.1.1 Mechanical Wheel Powder Feeder
3.5.1.2 Gravity‐Based Powder Feeder
3.5.1.3 Fluidized Bed Powder Feeder
3.5.1.4 Vibratory‐Based Powder Feeder
3.5.2 Powder Delivery Nozzles for DED
3.5.2.1 Lateral Nozzle
3.5.2.2 Coaxial Nozzle
3.5.3 Powder Bed Delivery and Spreading Mechanisms
3.5.4 Wire Feed System
3.5.5 Positioning Devices and Scanners in Laser‐Based AM
3.5.6 Print‐Head in Binder Jetting
3.6 CAD File Formats
3.6.1 CAD/CAM Software
3.7 Summary
References
4 Directed Energy Deposition (DED): Physics and Modeling of Laser/Electron Beam Material Processing and DED. Learning Objectives
4.1 Introduction
4.2 Laser Material Interaction and the Associated Significant Parameters to Laser AM
4.2.1 Continuous Versus Pulsed/Modulated Lasers
4.2.2 Absorption, Reflection, and Transmission Factors
4.2.3 Dependencies of Absorption Factor to Wavelength and Temperature
4.2.4 Angle of Incidence
4.2.5 Surface Roughness Effects
4.2.6 Scattering Effects
4.3 E‐beam Material Interaction
4.4 Power Density and Interaction Time for Various Heat Source‐based Material Processing
4.5 Physical Phenomena and Governing Equations During DED1
4.5.1 Absorption
4.5.2 Heat Conduction
4.5.3 Surface Convection and Radiation
4.5.4 Fluid Dynamics
4.5.4.1 Wetting Angles, Interfacial Free Energies, and Capillary Effects
4.5.5 Phase Transformation
4.5.6 Rapid Solidification
4.5.7 Thermal Stresses
4.5.8 Flow Field in DED with Injected Powder
4.5.8.1 Laminar Flow for LDED
4.5.8.2 Turbulent Flow for LDED
4.6 Modeling of DED
4.6.1 Analytical Modeling: Basics, Simplified Equations, and Assumptions
4.6.1.1 Analytical Solution of Heat Transfer Equation for Constant Extended Surface Heat
4.6.1.2 Analytical Model for an Instantaneous Point Source of Energy
4.6.1.3 Analytical Model for Continuous Point Source
4.6.1.4 Rosenthal's Equation
4.6.1.5 Adam's Equation
4.6.2 Numerical Models for DED3
4.6.3 Experimental‐based Models: Basics and Approaches4
4.7 Case Studies on Common Modeling Platforms for DED
4.7.1 Lumped Analytical Model for Powder‐Fed LDED5
4.7.2 Comprehensive Analytical Model for Powder‐Fed LDED (PF‐LDED)6
4.7.2.1 Model for Powder Spatial Distribution
4.7.2.2 Laser Beam and Gas–powder Stream Interaction
4.7.2.3 Thermal Conduction on the Substrate
4.7.2.4 Melt Pool and Deposited Track Geometry
4.7.2.5 Catchment Efficiency
4.7.2.6 Cooling and Solidification Rates7
4.7.2.7 Sample Results
4.7.3 Numerical Modeling of LDED: Heat Transfer Model8
4.7.3.1 Sample Results
4.7.4 Modeling of Wire‐Fed E‐beam DED (WF‐EDED)
4.7.5 A Stochastic Model for Powder‐Fed LDED9
4.8 Summary
References
Notes
5 Powder Bed Fusion Processes: Physics and Modeling. Learning Objectives
5.1 Introduction and Notes to Readers
5.2 Physics of Laser Powder bed Fusion (LPBF)
5.2.1 Heat Transfer in LPBF: Governing Equations and Assumptions
5.2.1.1 Volumetric Heat Source Models for LPBF
GMG Group
APG Group
5.2.1.2 Effective Thermal–Optical Material Properties in the Presence of Powder Particles
5.2.1.3 Effective Layer Thickness (ELT)
5.2.2 Fluid Flow in the Melt Pool of LPBF: Governing Equations and Assumptions
5.2.2.1 Marangoni Convection
5.2.2.2 Buoyancy and Electromagnetic Forces
5.2.2.3 Melt Pool Size and Shape: Keyhole Versus Conduction Modes
5.2.3 Vaporization and Material Expulsion: Governing Equations and Assumptions. 5.2.3.1 Evaporation Rate
5.2.3.2 Material Expulsion and Recoil Force
5.2.4 Thermal Residual Stresses: Governing Equations and Assumptions
5.2.5 Numerical Modeling of LPBF
5.2.5.1 Micro‐Scale Numerical Modeling Methods for LPBF
Molecular Dynamics Model
Discrete/Dynamic Element Model
Finite Element Model
5.2.6 Case Studies on Common LPBF Modeling Platforms
5.2.6.1 Melt Pool Size Estimation in LPBF1
Printed Track Surfaces: Experimental and Modeling Results
Prediction of Melt Pool Dimensions: Experimental and Modeling Results
5.2.6.2 Modeling of Residual Stress Induced During LPBF
5.3 Physics and Modeling of Electron Beam Additive Manufacturing
5.3.1 Electron Beam Additive Manufacturing Parameters
5.3.1.1 Electron Beam Parameters
5.3.1.2 Environmental Parameters
5.3.2 Emissions in Electron Beam Sources
5.3.2.1 Thermionic Emission
5.3.2.2 Field Emission
5.3.2.3 Schottky Emission
5.3.3 Mathematical Description of Free Electron Current
5.3.3.1 Lorentz Forces
5.3.3.2 Electron Acceleration
5.3.4 Modeling of Electron Beam Powder Bed Fusion (EB‐PBF) 5.3.4.1 Motivations for Modeling
5.3.4.2 Energy Transfer and Transformation
5.3.4.3 Navier–Stokes Equation
5.3.4.4 Numerical Methods for Solving Heat Transfer and Navier–Stokes Equation
Lattice Boltzmann method
Lattice Boltzmann Expansion for EB‐PBF
Melting and Solidification of Powder by the LB Method
Simulation of Powder Bed Particle Distribution by the LB Method
Additional Layers in the LB Method
Lattice Boltzmann's Solution Methods
Finite Element Methods
5.3.5 Case Studies
5.3.5.1 Micro‐ and Macro‐Scale Modeling of the EB Heat Source
5.3.5.2 Temperature Distribution During EB‐PBF
Heating and Cooling Rate
5.3.5.3 Stress Distribution During EB‐PBF
5.3.6 Summary
References
Note
6 Binder Jetting and Material Jetting: Physics and Modeling. Learning Objectives
6.1 Introduction
6.2 Physics and Governing Equations. 6.2.1 Droplet Formation
6.2.1.1 Material Properties and Dimensionless Numbers
6.2.1.2 Scaling Theories of Pinch‐off
6.2.2 Droplet–Substrate Interaction
6.2.3 Binder Imbibition
6.2.3.1 Geometrical Features of Liquid Bridge Among Particles
6.3 Numerical Modeling
6.3.1 Level‐Set Model1
6.3.2 Lattice Boltzmann Method
6.4 Summary
References
Note
7 Material Extrusion: Physics and Modeling. Learning Objectives
7.1 Introduction
7.2 Analytical Modeling of ME
7.2.1 Heat Transfer and Outlet Temperature
7.2.2 Flow Dynamics and Drop Pressure
7.2.3 Die Swell
7.2.4 Deposition and Healing
7.3 Numerical Modeling of ME
7.4 Summary
References
8 Material Design and Considerations for Metal Additive Manufacturing. Learning Objectives
8.1 Historical Background on Materials
8.2 Materials Science: Structure–Property Relationship
8.3 Manufacturing of Metallic Materials
8.4 Solidification of Metals: Equilibrium
8.5 Solidification in Additive Manufacturing: Non‐Equilibrium
8.6 Equilibrium Solidification: Theory and Mechanism
8.6.1 Cooling Curve and Phase Diagram
8.7 Non‐Equilibrium Solidification: Theory and Mechanism
8.8 Solute Redistribution and Microsegregation
8.9 Constitutional Supercooling
8.10 Nucleation and Growth Kinetics
8.10.1 Nucleation
8.10.2 Growth Behavior
8.11 Solidification Microstructure in Pure Metals and Alloys
8.12 Directional Solidification in AM
8.13 Factors Affecting Solidification in AM. 8.13.1 Cooling Rate
8.13.2 Temperature Gradient and Solidification Rate
8.13.3 Process Parameters
8.13.4 Solidification Temperature Span
8.13.5 Gas Interactions
8.14 Solidification Defects
8.14.1 Porosity
8.14.2 Balling
8.14.3 Cracking
8.14.3.1 Solidification Cracking
8.14.3.2 Intergranular Cracking
8.14.3.3 Reheat Cracking
8.14.3.4 Liquation Cracking
8.14.4 Lamellar Tearing
8.15 Post Solidification Phase Transformation
8.15.1 Ferrous Alloys/Steels
8.15.2 Al Alloys
8.15.3 Nickel Alloys/Superalloys
8.15.3.1 Inconel 625
8.15.3.2 Inconel 718
8.15.3.3 Stellite
8.15.4 Titanium Alloys
8.16 Phases after Post‐Process Heat Treatment. 8.16.1 Ferrous Alloys
8.16.2 Al Alloys
8.16.3 Ni Alloys
8.16.4 Ti Alloys
8.17 Mechanical Properties
8.17.1 Hardness
8.17.1.1 Hardness of AM‐Processed Ferrous Alloys
8.17.1.2 Hardness of AM‐Manufactured Al Alloys
8.17.1.3 Hardness of AM‐Manufactured Nickel Alloys
8.17.1.4 Hardness of AM‐Manufactured Ti Alloys
8.17.2 Tensile Strength and Static Strength
8.17.2.1 Tensile Behavior of AM‐Fabricated Ferrous Alloys
8.17.2.2 Tensile Behavior of AM‐Fabricated Al Alloys
8.17.2.3 Tensile Properties of AM‐Manufactured Ni Alloys
8.17.2.4 Tensile Properties of AM‐Manufactured Ti Alloys
8.17.3 Fatigue Behavior of AM‐Manufactured Alloys
8.17.3.1 Factors Influencing Fatigue Behavior in AM
8.17.3.2 Fatigue Performance of AM‐Manufactured Ferrous Alloys
8.17.3.3 Fatigue Behavior of AM‐Fabricated Al Alloy
8.17.3.4 Fatigue Property of AM‐Manufactured Nickel Alloys
8.17.3.5 Fatigue Behavior of Additive‐Manufactured Ti Alloy
8.18 Summary
References
9 Additive Manufacturing of Metal Matrix Composites. Learning Objectives
9.1 Introduction
9.2 Conventional Manufacturing Techniques for Metal Matrix Composites (MMCs)
9.3 Additive Manufacturing of Metal Matrix Composites (MMCs)
9.4 AM Challenges and Opportunities
9.5 Preparation of Composite Materials: Mechanical Mixing
9.6 Different Categories of MMCs
9.7 Additive Manufacturing of Ferrous Matrix Composites
9.7.1 316 SS‐TiC Composite
9.7.1.1 Solidification Phases of 316 SS‐TiC Composite: Theory of Fabrication
9.7.2 316 SS‐TiB2 Composite
9.7.3 H13–TiB2 Composite
9.7.4 H13–TiC Composite
9.7.5 Ferrous–WC Composite
9.7.6 Ferrous–VC Composites
9.8 Additive Manufacturing of Titanium‐Matrix Composites (TMCs)
9.8.1 Ti–TiC Composite
9.8.2 Ti–TiB Composites
9.8.2.1 Solidification Phases of Ti–TiB Composite: Theory of Fabrication
9.8.2.2 Formation Mechanism of Three‐Dimensional Quasi Continuous Network of TiB
9.8.3 Ti–Hydroxyapatite (Ti–HA) Composites
9.8.3.1 Solidification Phases of Ti–HA Composite: Theory of Fabrication
9.8.4 Ti‐6Al‐4V‐Metallic Glass (MG) Composites
9.8.5 Ti‐6Al‐4V + B4C Pre‐alloyed Composites
9.8.6 Ti‐6Al‐4V + Mo Composite
9.8.7 Structure and Properties of Different TMCs
9.9 Additive Manufacturing of Aluminum Matrix Composites
9.9.1 Al–Fe2O3 Composite
9.9.2 AlSi10Mg–SiC Composite
9.9.3 AlSi10Mg–TiC Composite
9.9.4 2024Al–TiB2 Composite
9.9.5 AlSi10Mg–TiB2 Composite
9.9.6 AA7075–TiB2 Composite
9.10 Additive Manufacturing of Nickel Matrix Composites
9.10.1 Inconel 625–TiC Composites
9.10.2 Inconel 625–TiB2 Composite
9.11 Factors Affecting Composite Property. 9.11.1 Mixing of Matrix and Reinforcing Elements
9.11.2 Size of Reinforcing Elements
9.11.3 Decomposition Temperature
9.11.4 Viscosity and Pore Formation
9.11.5 Volume of Reinforcing Elements and Pore Formation
9.11.6 Buoyancy Effects and Surface Tension Forces
9.12 Summary
References
10 Design for Metal Additive Manufacturing. Learning Objectives
10.1 Design Frameworks for Additive Manufacturing
10.1.1 Integrated Topological and Functional Optimization DfAM
10.1.2 Additive Manufacturing‐Enabled Design Framework
10.1.3 Product Design Framework for AM with Integration of Topology Optimization
10.1.4 Multifunctional Optimization Methodology for DfAM
10.1.5 AM Process Model for Product Family Design
10.2 Design Rules and Guidelines
10.2.1 Laser Powder Bed Fusion (LPBF)
10.2.2 Electron Beam Powder Bed Fusion (EB‐PBF)
10.2.3 Binder Jetting
10.2.4 Technologies Compared
10.3 Topology Optimization for Additive Manufacturing
10.3.1 Structural Optimization
10.3.2 Topology Optimization
10.3.2.1 Material Interpolation Functions
10.3.2.2 Sensitivity Analysis and Filtering
10.3.3 Design‐Dependent Topology Optimization
10.3.3.1 Review on Efforts to Tackle Multiobjective Thermomechanical Topology Optimization Problems
10.3.3.2 Thermomechanical Topology Optimization Based on Compliance Minimization
10.3.3.3 Thermal Stress Coefficient and Load
10.3.3.4 Thermomechanical Topology Optimization Problem Definition
10.3.4 Efforts in AM‐Constrained Topology Optimization
10.3.4.1 Overhang Minimization and Elimination
10.3.4.2 Self‐Supporting Constraint
10.3.4.3 Void Filling Constraint
10.3.4.4 Minimum Feature Thickness
10.3.4.5 Other AM Constraints
10.4 Lattice Structure Design
10.4.1 Unit Cell
10.4.2 Lattice Framework
10.4.3 Uniform Lattice
10.4.4 Conformal Lattices
10.4.5 Irregular/Randomized Lattices
10.4.6 Design Workflows for Lattice Structures
10.4.6.1 Hybrid Geometric Model
10.4.6.2 Combined Topology Optimization and Lattice Design for Constructing Functionally Graded Lattices
10.4.6.3 Relative Density Mapping (RDM) Method
10.5 Design for Support Structures
10.5.1 Principles that Should Guide Support Structure Design
10.5.2 Build Orientation Optimization
10.5.2.1 Build Orientation Optimization of an Arbor Press Framework
10.5.3 Support Structure Optimization
10.6 Design Case Studies
10.6.1 Redesign of an Aerospace Bracket to be Made by LPBF
10.6.2 Design and Development of a Structural Member in a Suspension Assembly Using EB Powder Bed Fusion
10.6.3 Binder Jetting of the Framework of a Partial Metal Denture
10.6.4 Redesign of a Crank and Connecting Rod
10.6.5 Redesign of a Mechanical Assembly
10.6.6 Solid‐Lattice Hip Prosthesis Design
10.7 Summary
References
11 Monitoring and Quality Assurance for Metal Additive Manufacturing. Learning Objectives
11.1 Why are Closed‐Loop and Quality Assurance Platforms Essential?[1]1
11.2 In-Situ Sensing Devices and Setups
11.2.1 Types of Sensors Used in Metal AM
11.2.1.1 Radiative Sensors
Cameras
Visible to NIR
NIR to LWIR
Inline Coherent Imaging (ICI)
X‐Ray Imaging
Photodiode
Pyrometer
11.2.1.2 Non‐Radiative
Acoustic Sensors
Thermocouple
Displacement and Stress–Strain Sensors
11.2.2 Mounting Strategies for In‐line Monitoring Sensors in Metal AM Setups
11.3 Commercially Available Sensors
11.3.1 LPBF Commercial Sensors
11.3.2 LDED Commercial Sensors
11.4 Signal/Data Conditioning, Methodologies, and Classic Controllers for Monitoring, Control, and Quality Assurance in Metal AM Processes
11.4.1 Signal/Data Conditioning and Controllers for Melt Pool Geometrical Analysis
11.4.2 Signal/Data Conditioning and Methodologies for Temperature Monitoring and Analysis
11.4.3 Signal/Data Conditioning and Methodologies for the Detection of Porosity
11.4.4 Signal/Data Conditioning and Methodologies for Detection of Crack and Delamination
11.4.5 Signal/Data Conditioning and Methodologies for Detection of Plasma Plume and Spatters
11.5 Machine Learning for Data Analytics and Quality Assurance in Metal AM
11.5.1 Supervised Learning
11.5.1.1 Classification
Support Vector Machine (SVM)
Neural Network (NN) and Deep Learning (DL)
Adaptive Neuro‐Fuzzy Inference System (ANFIS)
K‐Nearest Neighbors (KNN)
11.5.1.2 Regression Model
Gaussian Process (GP)
Neural Network (NN)
11.5.2 Unsupervised Learning
11.5.2.1 Clustering
K‐Means
Self‐Organized Map (SOM)
11.5.2.2 Data Reduction
Principal Component Analysis (PCA)
Singular Value Decomposition (SVD)
11.6 Case Study
11.6.1 Design of Experiments
11.6.2 In‐Situ Sensors and Quality Assurance Algorithm
11.6.3 Correlation Between CT Scan and Analyzed Data
11.7 Summary
References
Note
12 Safety. Learning Objectives
12.1 Introduction
12.2 Overview of Hazards
12.3 AM Process Hazards
12.4 Laser Safety in Additive Manufacturing1. 12.4.1 Laser Categorization
12.4.1.1 Class 1 Lasers
12.4.1.2 Class 2 Visible Lasers (400–700 nm)
12.4.1.3 Class 3A Lasers
12.4.1.4 Class 3B Lasers
12.4.1.5 Class 4 Lasers
12.4.2 Laser Hazards. 12.4.2.1 Eye Hazards
Details of Irradiation Effects on Eyes
Ultraviolet – B+C (100–315 nm)
Ultraviolet –A (315–400 nm)
Visible Light and Infrared‐A (400–1400 nm)
Infrared‐B (1400–3000 nm) and Infrared‐C (3000–10000 nm)
12.4.2.2 Laser Radiation Effects on Skin
12.4.2.3 Collateral Radiation
Ionizing Radiation
UV and Visible
Plasma Emissions
12.4.2.4 Electrical Hazards
Safety Guidelines
12.4.2.5 Chemical Hazards
12.4.2.6 Fire Hazards
12.4.2.7 Explosion Hazards
12.4.3 Eye Protection
12.4.4 Laser Protective Eyewear Requirements
12.5 Electron Beam Safety
12.6 Powder Hazards
12.6.1 Combustibility
12.7 Human Health Hazards
12.8 Comprehensive Steps to AM Safety Management. 12.8.1 Engineering Controls
12.8.2 Personal Protective Equipment
12.8.3 AM Guidelines and Standards
12.9 Summary
References
Note
Index
WILEY END USER LICENSE AGREEMENT
Отрывок из книги
Ehsan Toyserkani, Dyuti Sarker, Osezua Obehi Ibhadode, Farzad Liravi, Paola Russo, Katayoon Taherkhani
.....
Figure 1.14 (a) Dental crowns printed by LPBF
(Source: Courtesy of EOS [16]),
.....