EXTREMOPHILES as Astrobiological Models
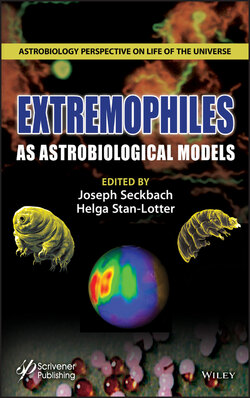
Реклама. ООО «ЛитРес», ИНН: 7719571260.
Оглавление
Группа авторов. EXTREMOPHILES as Astrobiological Models
Table of Contents
List of Illustrations
Tables
Guide
Pages
Extremophiles as Astrobiological Models
Preface. Foreword
Who and what is in this new book?
Conclusions
Acknowledgments
References
1. Volcanic Steam Vents: Life at Low pH and High Temperature
1.1 Introduction
1.2 Steam Cave and Vent Sites
1.3 Steam Cave and Vent Sample Collection
1.3.1 Steam Collection
1.3.2 Steam Deposit Collection
1.3.3 Steam and Steam Deposit Collection: Control Methods. Steam Controls
Steam Deposit Controls
1.4 Culture Isolation
1.5 Cell Structure of Isolates
1.6 Environmental Models
1.7 Conclusions
Acknowledgments
References
2. Rio Tinto: An Extreme Acidic Environmental Model of Astrobiological Interest
2.1 Introduction
2.2 Acidic Chemolithotrophy
2.3 Rio Tinto Basin
2.4 Biodiversity in the Tinto Basin
2.5 Tinto Basin Sedimentary Geomicrobiology
2.6 The Iberian Pyrite Belt Dark Biosphere
2.7 Methanogenesis in Non-Methanogenic Conditions
2.8 Rio Tinto: A Geochemical and Mineralogical Terrestrial Analog of Mars
2.9 Conclusions
References
3. Blossoms of Rot: Microbial Life in Saline Organic-Rich Sediments
3.1 Introduction
3.2 Overview of Saline Aquatic Systems
3.3 Prerequisites of Organic Carbon-Rich Sediment Genesis in Saline Lakes
3.4 Chemistry of Recent Organic Carbon-Rich Sediments in Saline Water Bodies
3.5 Microbial Life in Saline Sapropels
3.5.1 Higher-Order Microbial Taxonomy Detected in Modern Saline Sapropels
3.5.2 Anaerobic Organic Matter Degradation in Saline Organic Carbon-Rich Sediments
3.5.2.1 Hydrolysis of Biomacromolecules
3.5.2.2 Primary and Secondary Fermentation of Low Molecular Weight Molecules
3.5.2.3 Methanogenesis
3.5.2.4 Biological Sulfur Reduction and Oxidation
3.5.2.5 Nitrogen Cycling
3.5.3 Uncultured Microbial Diversity of Saline Sapropels and Its Putative Ecological Roles
3.5.3.1 Uncultured Bacteria
3.5.3.2 Uncultured Archaea
3.6 Relevance of Saline Sapropels
3.7 Concluding Remarks
Acknowledgments
References
4. The Haloarchaea of Great Salt Lake as Models for Potential Extant Life on Mars
4.1 The Great Salt Lake System in the Bonneville Basin. 4.1.1 A Significant Terminal Lake in North America
4.1.2 Great Salt Lake as an Extreme Environment
The North Arm is a Zone of Salt-Saturation
Ultraviolet Light Flux at Great Salt Lake
4.1.3 The Declining Elevation of Great Salt Lake
Ancient Lake Bonneville Becomes Great Salt Lake
The Modern Terminal Lake Shrinks and Swells
4.1.4 Pertinent Mineralogy of Great Salt Lake and the Bonneville Basin
4.2 The Transformation of an Ancient Wet Mars to a Modern Hostile Environment
4.2.1 Global Climatic Change on Mars
4.2.2 The Ancient Lacustrine Environments of Mars
4.2.3 Brine on the Martian Surface
4.2.4 Mars is a Hostile Environment for Life
Low and Unstable Temperature
Ultraviolet Radiation Flux
4.3 Life in Evaporitic Minerals on Earth
4.3.1 Formation of Halite and Gypsum Primary Crystals in Aquatic Environments
4.3.2 Haloarchaea May Be Entombed in Halite
4.3.3 Haloarchaea May Be Entombed in Gypsum
4.3.4 Haloarchaea Can Survive over Geologic Time in Fluid Inclusions
4.4 Great Salt Lake Haloarchaea
4.5 Haloarchaea Have Superpowers for Extreme Lifestyles
4.5.1 Haloarchaea May Show Versatility with Respect to Temperature and pH
4.5.2 Haloarchaea are Metabolically Versatile
4.5.3 Haloarchaea Are Adapted to High Salinity and Desiccating Conditions
4.5.4 Haloarchaea Are Resistant to Ultraviolet Radiation
4.5.5 Haloarchaea Are Resistant to Ionizing Radiation
4.5.6 Haloarchaea May Have Novel Nutrient Attainment Strategies for Dormancy Periods
4.5.7 Haloarchaea Are Resistant to Space Conditions
4.6 Extant or Extinct Haloarchaea on Mars?
4.6.1 Haloarchaea as Extant Life
4.6.2 Haloarchaea as Extinct Life
Nucleic Acids as Biosignatures
Proteins as Biosignatures
Carbohydrates as Biosignatures
Lipids as Biosignatures
Contamination and Biosignatures
4.7 Conclusions and Insights
Acknowledgments
References
5. Arsenic-and Light Hydrocarbon-Rich Hypersaline Soda Lakes and Their Resident Microbes as Possible Models for Extraterrestrial Biomes
5.1 Introduction
First, however, a personal digression
5.2 Mars
5.3 Enceladus
5.4 Titan
References
6. Antarctic Bacteria as Astrobiological Models
Abbreviations
6.1 Introduction
6.2 Antarctica as an Analogous Environment for Astrobiology
6.2.1 Temperature
6.2.2 Soil as a Life-Supporting Medium
6.2.3 Soil Salinity
6.3 Astrobiological Environments of Interest
6.4 Bacterial Adaptations to Extreme Environments as Analogues for Astrobiology
6.4.1 Psychrophiles
6.5 Antarctic Bacteria as Analogues for Astrobiology
6.6 Endemic Antarctic Bacteria used in Astrobiology
6.7 Cosmopolitan Bacteria Found in Antarctica and used in Astrobiology
6.8 Conclusion
References
7. Extremophilic Life in Our Oceans as Models for Astrobiology
7.1 Introduction
7.2 Southern Ocean Ecosystem: West Antarctic Peninsula Region
7.3 Sea Ice Decline in WAP and Ice Shelf Collapse in Amundsen Sea
7.4 Deoxygenation Leading toward Hypoxic Zone in Amundsen Sea
7.5 Microbial Extremophiles in Southern Ocean
7.6 Chemosynthetic Abyssal Ecosystems. 7.6.1 Methane Cold Seep Deep-Sea Communities in the Abyss
7.6.2 Are Hydrothermal Vents Abyssal Gold Mines?
7.7 Hydrothermal Activity in Hrad Vallis on Mars
7.8 Why Chemosynthetic Ecosystems Remind Us of Environmental Conditions When Life Originated in the Universe
7.9 Ultra-Abyssal Ecosystem: Puerto Rico Trench
7.10 Affiliations of Abyssal Life to Astrobiology: Some Perspectives
7.11 Can We Find Protozoans Such as Xenophyophores on Other Planets?
7.12 Barophilic Organisms in the Deep-Sea
Acknowledgments
References
8. Challenging the Survival Thresholds of a Desert Cyanobacterium under Laboratory Simulated and Space Conditions
8.1 Introduction
8.2 Endurance of Chroococcidiopsis Under Air-Drying and Space Vacuum
8.3 Endurance of Chroococcidiopsis Under Laboratory Simulated and Space Radiation
8.4 The Use of Chroococcidiopsis’s Survival Thresholds for Future Astrobiological Experiments
Acknowledgments
References
9. Lichens as Astrobiological Models: Experiments to Fathom the Limits of Life in Extraterrestrial Environments
9.1 Introduction
9.2 Survival of Lichens in Outer Space
9.3 Space Environment: Relevance in Space Science
9.3.1 Space Radiation
9.3.2 Temperature
9.3.3 Microgravity
9.4 Biological Effects of Space. 9.4.1 Extraterrestrial Solar UV Radiation
9.4.2 Cosmic Radiation
9.4.3 Space Vacuum
9.4.4 Microgravity
9.5 Current and Past Astrobiological Facilities for Experiments with Lichens
9.6 Space Experiments with Lichens. 9.6.1 The BIOPAN Experiments: Exposure to Outer Space Conditions. Lichens Experiment
LITHOPANSPERMIA and LITHOPANSPERMIA/STONE Experiments
9.6.2 EXPOSE Facility On Board the ISS: Experiments at Space- and Mars-Like Conditions
LIFE Experiment
BIOMEX Experiment
9.7 Simulation Studies
9.8 Summary and Conclusions
9.9 Future Possibilities and Recommendations
References
10. Resistance of the Archaeon Halococcus morrhuae and the Biofilm-Forming Bacterium Halomonas muralis to Exposure to Low Earth Orbit for 534 Days
Introduction
10.2 Material and Methods. 10.2.1 Cultivation
10.2.2 UV-C Radiation and Desiccation Resistance
10.2.3 Sample Preparation for Exposure
10.2.4 Pre-Flight Ground Tests
10.2.5 Flight Preparation and Mission Ground Reference (MGR) Preparation
10.2.6 Mission EXPOSE-R2 – Flight Parameters and Conditions
10.2.7 Survival
10.2.8 Stability of Genomic DNA
10.2.9 Integrity of 16S rRNA Gene
Results
10.3.1 Survival EVT/SVT
10.3.2 Survival and Genetic Integrity Following Space Flight
10.4 Discussion
Acknowledgments
References
11. The Amazing Journey of Cryomyces antarcticus from Antarctica to Space
11.1 Introduction
11.2 The McMurdo Dry Valleys
11.3 Cryptoendolithic Communities
11.4 The Black Microcolonial Yeast-like Fungus Cryomyces antarcticus
11.5 The Polyextremotolerance of Cryomyces antarcticus
11.6 Cryomyces antarcticus and its Resistance to Radiation in Ground-Based Simulated Studies
11.7 C. antarcticus and its Resistance to Actual Space Exposure in Low Earth Orbit
11.7.1. LIFE Experiment on EXPOSE-E on Board the International Space Station
11.7.2. BIOMEX Experiment on EXPOSE-R2 on Board the International Space Station
11.8 Conclusion
11.9 Future Perspectives
Acknowledgments
References
12. Tardigrades – Evolutionary Explorers in Extreme Environments
12.1 Introduction
12.2 The Evolutionary Transition Towards Cryptobiotic Adaptations in Tardigrades
12.3 Cryptobiosis as an Evolutionary Adaptive Strategy
12.4 Defining Life in Cryptobiotic Animals
12.5 A Resilience Approach to the Cryptobiotic State
12.6 Molecular Mechanisms for Structural Stability in the Dry State
12.7 Tardigrades as Astrobiological Models
12.8 Tardigrades – Extremotolerants or Extremophiles?
Acknowledgments
References
13. Spore-Forming Bacteria as Model Organisms for Studies in Astrobiology
13.1 Introduction
13.2 Historical Beginnings
13.2.1 Evolution and the Origin of Life
13.2.2 Panspermia
13.2.3 Microbiology
13.2.4 Bacterial Spores
13.3 Revival of Lithopanspermia
13.4 Testing Lithopanspermia Experimentally
13.4.1 Impact-Mediated Launch
13.4.2 Transit Through Interplanetary Space
13.4.3 Atmospheric Entry and Deposition
13.5 Lithopanspermia, Spores, and the Origin of Life
13.6 Interstellar Lithopanspermia
13.7 Humans as Agents of Panspermia
13.8 Survival and Growth of Spores in the Mars Environment
Acknowledgments
References
14. Potential Energy Production and Utilization Pathways of the Martian Subsurface: Clues from Extremophilic Microorganisms on Earth
14.1. Introduction
14.2. Energy Sources
14.2.1 Hydrogen
Extremophiles Using Hydrogen
14.2.2 Methane
Extremophiles Using Methane
14.2.3 Iron
Extremophiles Using Iron
14.2.4 Sulfur and Associated Compounds
Extremophiles Using Sulfur
14.2.5 Other Potential Energy Sources. Nitrogen
Manganese
Perchlorate
Organics
Special Mention: Oxygen
14.3. Conclusion
References
15. Origin of Initial Communities of Thermophilic Extremophiles on Earth by Efficient Response to Oscillations in the Environment
15.1 Introduction
15.2 Required Conditions for the Origin of Life: Necessity of Rapid-Frequency Oscillations of Parameters
15.3 Parameters of the Environment for the Origin of Life
15.4 Formation of Prebiotic Microsystem Clusters and Their Conversion into Primary Communities of Thermophilic Extremophiles
15.5 Theoretical and Experimental Verification of the Proposed Approach
15.6 Conclusion
References
16. Extremophiles and Horizontal Gene Transfer: Clues to the Emergence of Life
16.1 Introduction
16.2 T-LUCAs, LUCAs and Progenotes
16.3 Prebiotic World and T-LUCA
16.4 Emergence of LUCA
16.5 Chemical Composition of LUCA
16.6 Emergence of Cellular Life Forms
16.7 Evidence for the Earliest Cellular Life Forms
16.8 The Hypotheses: Genetic First vs. Metabolism First
16.9 Extremophiles
16.10 The Viral Connection to the Origin of Life
16.11 Horizontal Gene Transfer (HGT)
16.12 Mechanisms of HGT
16.13 Clues to the Origins of Life and a Phylogenetic Tree
16.14 Conclusion
Acknowledgment
References
17. What Do the DPANN Archaea and the CPR Bacteria Tell Us about the Last Universal Common Ancestors?
17.1 Introduction
17.2 The Discovery of DPANN and CPR
17.3 Common Features of CPR and DPANN
17.4 LUCA and the Deep-Rootedness of CPR and DPANN
17.5 Short Branches, Deep Branches and Multiple LUCAs
17.6 Viruses: LUCA without ‘Cellular’
References
18. Can Biogeochemistry Give Reliable Biomarkers in the Solar System?
Abbreviations
18.1 Evidence of Life in the Solar System
18.2 Extremophiles on Earth
18.3 Extremophiles in Low Orbits Around the Earth
18.4 Have There Been Extremophiles on the Moon?
18.5 Have There Been Extremophiles on Mars?
18.6 Europa is a Likely Location for an Extremophilic Ecosystem
18.7 Are There Other Environments for Extremophiles in the Solar System? 18.7.1 Titan
18.7.2 Enceladus
18.7.3 Triton, Icy Moons, Pluto and Charon
18.8 Are There Environments for Extremophiles on Exoplanets?
References
Index
WILEY END USER LICENSE AGREEMENT
Отрывок из книги
Scrivener Publishing
100 Cummings Center, Suite 541J
.....
2.21. Chapelle, F.H., O´Nelly, K., Bradley, P.M., Methé, B.A., Ciufo, S.A., Knobel, L.L., Lovley, D.R., A hydrogen-based subsurface microbial community dominated by methanogens. Nature, 415, 312–314, 2002.
2.22. Colín-García, M., Kanawati, B., Harir, M., Schmidt-Kopplin, P., Amils, R., Parro, V., García, M., Fernández-Remolar, D., Detection of peptidic sequences in the ancient acidic sediments of Río Tinto, Spain. Orig. Life Evol. Biosph., 41, 523–527, 2011.
.....