Position, Navigation, and Timing Technologies in the 21st Century
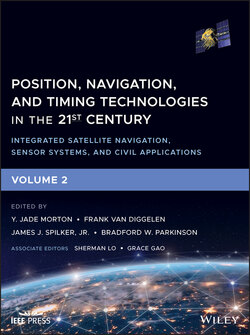
Реклама. ООО «ЛитРес», ИНН: 7719571260.
Оглавление
Группа авторов. Position, Navigation, and Timing Technologies in the 21st Century
Table of Contents
List of Tables
List of Illustrations
Guide
Pages
Position, Navigation, and Timing Technologies in the 21st Century. Integrated Satellite Navigation, Sensor Systems, and Civil Applications
Preface
Note
Contributors
35 Overview of Volume 2: Integrated PNT Technologies and Applications
35.1 Generalized Navigation Framework
35.1.1 What Is a Navigation Sensor?
35.2 Summary of Content of Volume 2
36 Nonlinear Recursive Estimation for Integrated Navigation Systems
36.1 Introduction
36.1.1 Notation
36.2 Linear Estimation Foundations
36.2.1 Typical Recursive Estimation Framework
36.3 Nonlinear Filtering Concepts
36.3.1 Effects of Nonlinear Operations on Random Processes – Breaking Up with Gauss
36.3.2 Gaussian Sum Filters
36.3.2.1 Multiple Model Adaptive Estimation
36.3.3 MMAE Example – Integer Ambiguity Resolution
36.3.4 Particle Filters
36.3.5 Grid Particle Filtering
36.3.6 Grid Particle Filter Example Application
36.3.7 Sampling Particle Filter (SIS/SIR)
36.3.8 Sequential Importance Sampling Recursive Estimator
36.3.9 Sampling Particle Filter Demo
36.3.10 Strengths and Weaknesses of Approaches
36.4 Summary and Conclusions
References
37 Overview of Indoor Navigation Techniques
37.1 Introduction
37.2 Overview of Technical Terms
37.3 Performance Metrics
37.4 Indoor Localization Signal Classification
37.4.1 Infrared Radiation (IR) and Visible Light
37.4.2 RF Signals
37.4.2.1 Personal and Local Area Networks
37.4.2.2 Broadcast and Wide Area Networks
37.4.2.3 Challenges
37.4.3 Ultrasound
37.4.4 Inertial and Mechanical
37.4.5 Other Signals
37.5 Indoor Localization Techniques
37.5.1 Triangulation
37.5.1.1 Angle‐Based Methods
37.5.1.2 Time‐Based Methods
37.5.1.3 Signal‐Property‐Based Methods
37.5.2 Fingerprinting
37.5.3 Proximity
37.5.4 Dead Reckoning
37.5.5 Map Matching
37.5.6 Hybrid Techniques
37.5.6.1 GPS‐Based Techniques
37.5.6.2 Techniques Fusing RF Signals with Dead Reckoning
37.5.6.3 Techniques Fusing RF Signals with Other Signals
37.5.6.4 Techniques Fusing Dead Reckoning with Non‐RF Signals
37.6 Open Research Issues
References
38 Navigation with Cellular Signals of Opportunity
38.1 Introduction
38.2 Overview of Cellular Systems
38.3 Clock Error Dynamics Modeling
38.4 Navigation Frameworks in Cellular Environments
38.4.1 Mapper/Navigator Framework
38.4.2 Radio SLAM Framework
38.5 Navigation with Cellular CDMA Signals
38.5.1 Forward Link Signal Structure
38.5.1.1 Modulation of Forward Link CDMA Signals
38.5.1.2 Pilot Channel
38.5.1.3 Sync Channel
38.5.1.4 Paging Channel
38.5.1.5 Transmitted Signal Model
38.5.1.6 Received Signal Model
38.5.2 CDMA Receiver Architecture
38.5.2.1 Correlation Function
38.5.2.2 Acquisition
38.5.2.3 Tracking
38.5.2.4 Message Decoding
38.5.3 Code Phase Error Analysis
38.5.3.1 Discriminator Statistics
38.5.3.2 Closed‐Loop Analysis
38.5.4 Cellular CDMA Navigation Experimental Results
38.5.4.1 Pseudorange Analysis
38.5.4.2 Ground Vehicle Navigation
38.5.4.3 Aerial Vehicle Navigation
38.6 Navigation with Cellular LTE Signals
38.6.1 LTE Frame and Reference Signal Structure
38.6.1.1 Frame Structure
38.6.1.2 Timing Signals
38.6.1.3 Received Signal Model
38.6.2 LTE Receiver Architecture
38.6.2.1 Acquisition
38.6.2.2 System Information Extraction
38.6.2.3 Tracking
38.6.2.4 Timing Information Extraction
38.6.3 Code Phase Error Analysis
38.6.3.1 Coherent DLL Tracking
38.6.3.2 Non‐Coherent DLL Tracking
38.6.3.3 Code Phase Error Analysis in Multipath Environments
38.6.4 Cellular LTE Navigation Experimental Results
38.6.4.1 Pseudorange Analysis
38.6.4.2 Ground Vehicle Navigation
38.6.4.3 Aerial Vehicle Navigation
38.7 BTS Sector Clock Bias Mismatch
38.7.1 Sector Clock Bias Mismatch Detection
38.7.2 Sector Clock Bias Discrepancy Model Identification
38.7.3 PNT Estimation Performance in the Presence of Clock Bias Discrepancy
38.8 Multi‐Signal Navigation: GNSS and Cellular
38.8.1 DOP Reduction
38.8.2 GPS and Cellular Experimental Results. 38.8.2.1 Ground Vehicle Navigation
38.8.2.2 Aerial Vehicle Navigation
38.9 Cellular‐Aided INS
38.9.1 Radio SLAM with Cellular Signals
38.9.2 Simulation Results
38.9.3 Experimental Results
References
39 Position, Navigation and Timing with Dedicated Metropolitan Beacon Systems
39.1 Metropolitan Beacon System (MBS)
39.1.1 System Description
39.1.2 Signal Description
39.1.3 Comparison of MBS Signal with GNSS Signals
39.1.4 Receiver Architecture
39.1.4.1 Signal Dynamic Range and Gain Control
39.1.4.2 Signal Acquisition, Tracking, and Ranging
39.1.4.3 Position Calculation
39.1.5 Assisted Mode of MBS
39.1.6 MBS System for Time and Frequency Synchronization
39.1.7 Standardization (3GPP, OMA) and MBS Call Flows
39.1.8 Performance Results
39.1.9 Conclusion
References
40 Navigation with Terrestrial Digital Broadcasting Signals
40.1 PNT Mechanisms with Broadcasting Signals
40.2 Representative Terrestrial Digital Broadcasting Signals
40.2.1 Acquisition and Tracking of ATSC‐8VSB Signals for Timing and Ranging
40.2.2 Acquisition and Tracking of DVB‐T Signals for Timing and Ranging
40.2.3 ISDB‐T Signals for Timing and Ranging
40.2.4 DTMB Signals for Timing and Ranging
40.2.5 Next‐Gen ATSC 3.0 Signals for Timing and Ranging
40.3 Pseudorange Measurements from Broadcasting Signals
40.4 Practical Issues and Search for Solutions
40.4.1 Analysis of Geometry Effect on Positioning Performance
40.4.2 Radio Dead Reckoning with Mixed SOOP
40.4.3 Toward Practical Robust Operations
References
41 Navigation with Low‐Frequency Radio Signals
41.1 Introduction
41.2 A Brief History of Very Low‐Frequency (VLF) and LF PNT
41.3 Loran‐C and eLoran Signal in Space Definition
41.3.1 Historical Loran‐C coverage
41.3.2 Pulse Shape and Cycle Identification
41.3.3 System Timing and Phase Code
41.3.4 Data Broadcast
41.4 Enhanced Loran (eLoran) Transmission. 41.4.1 Transmission Infrastructure
41.4.2 Transmitting Antennas
41.4.3 Transmission Synchronization
41.5 LF Propagation
41.5.1 Sky‐Wave versus Ground‐Wave Propagation
41.5.1.1 Ground‐Wave Tracking
41.5.2 Ground‐Wave Propagation: PF, SF, and ASF
41.5.2.1 Spatial ASF Variations
41.5.2.1.1 ASF Modeling
41.5.2.1.2 ASF Measurements
41.5.2.1.3 Combination of Measured and Modeled ASFs
41.5.2.1.4 ASF Measurement and Correction by Integrated eLoran‐GNSS Receiver
41.5.2.2 Temporal ASF Variations
41.5.3 Re‐Radiation
41.5.3.1 Response of E‐Field Antenna to Re‐Radiation
41.5.3.2 Response of H‐Field Antenna to Re‐Radiation
41.5.3.3 Re‐Radiation Mitigation
41.5.3.4 Implications of Re‐Radiation on Spatial and Differential ASF Corrections
41.6 Noise and Interference
41.6.1 Atmospheric Noise
41.6.2 Precipitation Static
41.6.3 Man‐Made Interference. 41.6.3.1 Continuous Wave Interference
41.6.3.2 Local Interference
41.6.4 Self‐Interference: CRI
41.6.4.1 CRI Mitigation by Receiver Processing
41.6.4.2 CRI Mitigation by System Redesign
41.6.4.2.1 Optimal GRI Selection
41.6.4.2.2 New Phase Code
41.6.4.2.3 Fewer Chains
41.6.4.2.4 Complete Overhaul of Station Pulse Patterns
41.7 Receiver Design
41.7.1 eLoran Receiver Design: Signal Reception and Conditioning
41.7.1.1 LF Antenna: E‐Field or H‐Field
41.7.1.1.1 Antenna Response to Re‐Radiation
41.7.1.1.2 Signal Penetration Indoors
41.7.1.1.3 P‐Static Sensitivity
41.7.1.1.4 Sensitivity to Local Interference
41.7.1.1.5 Antenna Noise Performance
41.7.1.2 E‐Field Antenna Design
41.7.1.2.1 E‐Field Antenna Grounding
41.7.1.3 H‐Field Antenna Design
41.7.1.3.1 H‐Field Antenna Sensing Element
41.7.1.3.2 Antenna Topology
41.7.1.3.3 Noise Performance
41.7.1.4 H‐Field Antenna Radiation Pattern
41.7.1.4.1 Quadrature Addition
41.7.1.4.2 Electronic Beam Steering
41.7.1.4.3 Electronic Compass Functionality
41.7.1.5 Heading‐Dependent H‐Field Antenna Errors
41.7.1.5.1 Parasitic E‐Field Susceptibility of H‐Field Antenna
41.7.1.5.2 Tuning Errors in a Resonant H‐Field Antenna. 41.7.1.5.2.1 Tuning Differences
41.7.1.5.2.2 Tuning Variations
41.7.1.5.3 Cross‐Talk
41.7.2 eLoran Receiver Design – Signal Tracking, Correction, and Position Determination
41.7.2.1 Signal Acquisition
41.7.2.2 Signal Tracking
41.7.2.2.1 Cycle Identification and Ground‐Wave Tracking
41.7.2.3 Data Demodulation
41.7.2.4 ASF Correction
41.7.2.5 Position Calculation
41.8 Loran Performance: Past, Present, and Future. 41.8.1 Legacy System Performance: Loran‐C
41.8.2 Next‐Generation Performance: Current eLoran
41.8.3 eLoran for Maritime Users
41.8.4 eLoran for Land‐Mobile Users
41.8.5 eLoran for Aviation Users
41.8.6 eLoran for Timing, Frequency, and Phase Users
41.8.7 eLoran for Location‐Based Users
41.8.8 eLoran for Precision Users
41.8.9 eLoran for Data Users
41.9 Potential of Future LF Radio Navigation Systems
References
42 Adaptive Radar Navigation
42.1 A History of Radar Localization
42.2 Modern Radar Localization
42.2.1 Terrain‐Aided Navigation Systems
42.2.2 Radar Velocity‐Aiding and Odometry
42.2.3 Tracked Object Relative Positioning
42.3 Radar Signal Processing. 42.3.1 Overview
42.3.2 Waveform Selection
42.3.3 Effects of Bandwidth and Modulation on Navigation Performance
42.3.4 Coherent Modulation/Demodulation
42.4 SAR Processing Methods
42.4.1 Polar Format Algorithm
42.4.2 Backprojection
42.5 UWB‐OFDM Case Study
42.5.1 Signal Generation and Transmission. 42.5.1.1 Signal Waveform Selection
42.5.1.2 Signal Properties
42.5.2 Navigation Algorithm Overview
42.5.3 Feature Extraction (Pulse Compression)
42.5.4 Feature Detection and Tracking
42.5.4.1 GNN Data Association
42.5.4.2 M/N Detector
42.5.4.3 Stochastic Extensions
42.5.5 INS Processing
42.5.6 Navigation Filter
42.5.6.1 Kalman Filter
42.5.6.2 Extended Kalman Filter
42.5.6.3 State Model
42.5.6.4 Dynamics Model
42.5.6.5 Measurement Model
42.5.6.6 EKF Initialization
42.5.7 Experimental Performance Analysis. 42.5.7.1 Signal Generation
42.5.7.2 System Results
42.5.7.3 SAR Navigation Results
42.6 Conclusion
References
43 Navigation from Low Earth Orbit: Part 1: Concept, Current Capability, and Future Promise
43.1 Introduction
43.1.1 Early Satellite Navigation
43.1.2 Big and Broadband LEOs
43.2 Background
43.2.1 Signal‐to‐Noise Ratio
43.2.2 Mean Motion
43.2.3 Satellite Footprint and Coverage
43.2.4 LEO Versus MEO Summary
43.3 LEOs in Navigation Today
43.3.1 Signal Strength in Challenging Environments
43.3.2 Indoor Time Transfer
43.3.3 Indoor Positioning
43.4 LEOs in Navigation Tomorrow
43.4.1 Position Accuracy
43.4.2 Geometry
43.4.3 User Range Error
43.4.3.1 Clock
43.4.3.2 Orbit
43.4.4 Radiation
43.5 Conclusion
References
43 Navigation from Low‐Earth Orbit: Part 2: Models, Implementation, and Performance
43.6 Introduction
43.7 LEO Satellite Pseudorange, Carrier Phase, and Doppler Measurement Model
43.7.1 Pseudorange Measurement Model
43.7.2 Doppler Measurement Model
43.7.3 Carrier‐Phase Measurement Model
43.8 LEO Satellite Orbital Dynamics Model
43.9 Navigation Error Sources
43.9.1 LEO Satellite Position and Velocity Error
43.9.2 LEO Satellite Clock Errors
43.9.3 Ionospheric and Tropospheric Errors
43.10 Overview of Orbcomm LEO Satellite Constellation
43.10.1 Orbcomm System Overview
43.10.2 Orbcomm Space Segment
43.10.3 Orbcomm Downlink Signals
43.10.4 Orbcomm Receiver Design
43.11 Overview of Starlink LEO Satellite Constellation
43.11.1 Proposed Starlink Constellation
43.11.2 Signal Information
43.12 Carrier‐Phase Differential Navigation with LEO Satellite Signals
43.12.1 Framework Formulation
43.12.2 Batch Navigation Solution
43.13 STAN: Simultaneous Tracking and Navigation with LEO Satellites’ Signals
43.13.1 EKF State Vector and Dynamics Model. 43.13.1.1 EKF State Vector
43.13.1.2 Vehicle Kinematics Model
43.13.1.3 LEO Satellite Dynamics Model
43.13.2 IMU Measurement Model and EKF Prediction
43.13.3 Receiver Measurement Model and EKF Update
43.14 Dilution of Precision Analysis
43.15 Simulation Results
43.15.1 Stand‐alone Navigation Solution with LEO Satellite Signals
43.15.2 LEO‐Aided INS STAN
43.15.2.1 UAV Simulation with Globalstar, Orbcomm, and Iridium LEO Constellations
43.15.2.2 UAV Simulation with the Starlink LEO Constellation with Periodically Transmitted LEO Satellite Positions
43.16 Experimental Results
43.16.1 Stand‐alone Navigation Solution with LEO Satellite Signals
43.16.2 CD–LEO Navigation Results
43.16.3 LEO‐Aided INS STAN
43.16.3.1 Ground Vehicle
43.16.3.2 Aerial Vehicle
References
44 Inertial Navigation Sensors
44.1 Introduction
44.2 Inertial Navigation Performance
44.2.1 Initial Condition Errors
44.2.2 Accelerometer Errors
44.2.3 Gyroscope Errors
44.3 IMU Performance Classes
44.4 Accelerometer Taxonomy
44.4.1 Unrestrained Proof Mass
44.4.2 Atomic Accelerometers
44.4.3 Spring Restraint
44.4.4 Pendulous Flexure
44.4.5 Vibrating Beam Accelerometer
44.4.6 Force‐Rebalanced Accelerometer
44.4.7 Inertial Rebalance Accelerometer
44.5 Gyroscopes
44.5.1 Taxonomy
44.5.2 Angular Momentum – Rotating Mass
44.5.3 Electrostatic Gyroscopes
44.5.4 Dynamically Tuned Gyroscopes
44.5.5 Nuclear Magnetic Resonance Gyroscopes
44.5.6 Coriolis
44.5.7 Hemispherical Resonator Gyroscope
44.5.8 Sagnac Effect
44.5.9 Interferometric Optical Rotation Sensors
44.5.10 Ring Laser Gyroscopes
44.5.11 Passive Ring Resonator Gyroscopes
44.5.12 Atomic Gyroscopes
44.6 Conclusion
References
Notes
45 MEMS Inertial Sensors
45.1 Introduction to Micro‐Electromechanical Systems (MEMS) Inertial Sensors
45.1.1 History
45.1.2 Silicon Manufacturing and Supply Chain
45.2 MEMS Accelerometers
45.2.1 Common Accelerometer Types
45.2.1.1 Piezoresistive
45.2.1.2 Capacitive
45.2.1.3 Thermal
45.2.2 Commercial Availability
45.2.3 Future Developments
45.3 MEMS Gyroscopes
45.3.1 Operating Principles
45.3.2 Common Gyroscope Types
45.3.2.1 Silicon Tuning Fork
45.3.2.2 Quartz Tuning Fork
45.3.2.3 Vibratory Ring
45.3.2.4 Bulk Acoustic Wave
45.3.3 Commercial Availability
45.3.4 Future Developments
45.4 MEMS IMUs
45.4.1 Architecture
45.4.2 Commercial Availability
45.5 Using MEMS Inertial Sensors in Navigation Solutions
45.5.1 Business Considerations
45.5.2 Sensor Selection
45.5.3 Sensor Performance Specifications
References
Notes
46 GNSS‐INS Integration: Part 1: Fundamentals of GNSS‐INS Integration
46.1 Main Principles of Inertial Navigation
46.2 Inertial Error Propagation
46.2.1 One‐Dimensional Case
46.2.2 Sensor Error Models for Three‐Dimensional Case
46.2.3 Error Propagation Through Attitude Computation
46.2.4 Error Propagation Through Coordinate Transformation
46.2.5 Error Propagation Through Integration
46.2.6 Bringing It All Together
46.3 Loose Integration: Solution‐Domain Sensor Fusion
46.3.1 Position Updates
46.3.2 Attitude Updates
46.3.3 Example Simulation Scenario
46.4 Tight Integration: Measurement‐Domain Sensor Fusion
46.4.1 Pseudorange Updates
46.4.2 Carrier‐Phase Updates
46.5 Deep Integration: Sensor Fusion at the Signal Processing Level
46.5.1 Example Implementation with Long Coherent Integration of GPS Signals
46.5.2 Computation of Dynamic Reference Trajectory
46.5.3 Error‐State Estimation
46.5.4 Treatment of Navigation Data Bits
46.6 Implementation Case Studies
46.6.1 GNSS/INS Integration for Navigation in Urban Environments
46.6.1.1 Measurement Synchronization
46.6.1.2 Data Quality Monitoring
46.6.1.3 Error Reset
46.6.1.4 Test Scenario
46.6.1.5 Test Results for Mid‐Grade MEMS IMU
46.6.1.6 Test Results for Consumer‐Grade MEMS IMU
46.6.2 Deep GPS/INS Integration for Navigation Under Dense Foliage
References
46 GNSS‐INS Integration: Part 2: GNSS/IMU Integration Using a Segmented Approach
46.7.1 Integration Approach. 46.7.1.1 Role of the IMU Reappraised
46.7.1.2 Basis for Key Steps
46.7.1.3 Specific Topology and Advantages
46.7.2 Algorithms
46.7.2.1 Position Incrementing
46.7.2.2 Velocity Incrementing
46.7.2.3 Attitude Incrementing
46.7.3 Error and Covariance Propagation
46.7.4 Measurement Differencing
46.7.4.1 Differencing Across Satellites
46.7.4.2 Differencing Across Receivers
46.7.4.3 Differencing Across Time
46.7.4.4 Correlations
46.7.5 One Second Change in Carrier Phase
46.7.6 Formation of Residuals
46.7.7 Formation of Sensitivities
46.7.8 Integrity Testing
46.7.9 GPS/INS Flight Test
46.7.9.1 Time Histories
46.7.9.2 Data‐Edit Performance
A. Appendix
References
47 Atomic Clocks for GNSS
47.1 Introduction
47.2 Basic Concepts. 47.2.1 Components of an Atomic Clock
47.2.2 Terminology
47.2.3 Characterizing Clock Performance
47.3 GNSS Space Clocks
47.3.1 Rb Atomic Frequency Reference (AFR)
47.3.2 Cs Thermal Beam AFR
47.3.3 H‐Maser AFR
47.4 Advanced Atomic Clocks for Future Use in Space
47.4.1 Advanced Microwave Space Clocks
47.4.2 Prospects for Optical Atomic Clocks in Space
47.5 Einstein’s Relativity for Clocks near Earth – Brief Summary
47.6 Atomic Clocks on Earth Supporting GNSS. 47.6.1 GNSS Master Clocks
47.7 National Standards Laboratories
47.8 AFRs for GNSS Receivers
47.9 Chip Scale Atomic Clocks (CSACs)
Acknowledgments
References
48 Positioning Using Magnetic Fields
48.1 Introduction
48.2 Magnetic Field Sources
48.2.1 Earth’s Core Magnetic Field
48.2.2 Earth’s Crustal Magnetic Field
48.2.3 Space Weather Magnetic Fields
48.2.4 Man‐Made Magnetic Fields
48.3 Magnetic Measurements and Instruments
48.3.1 Vector Instruments
48.3.2 Intensity Sensors
48.3.3 Gradient and Tensor Measurements
48.4 Magnetometer Calibration Approaches
48.4.1 Aircraft Effect Calibration
48.4.2 Calibrating Fluxgate Magnetometers
48.5 Absolute Positioning Using Magnetic Fields
48.5.1 Indoors
48.5.2 Ground Vehicles
48.5.2.1 AFIT Route Test Results
48.5.2.2 Neighborhood Route Test Results
48.5.2.3 Large Route Test Results
48.5.3 Aerial
48.5.3.1 Magnetic Anomaly Maps
48.5.3.2 The Measurement Equation
48.5.3.3 Modeling the Temporal Variations
48.5.3.4 Flight Testing of Magnetic Field Navigation
48.5.3.5 Practical Issues with Aircraft Magnetic Field Navigation
Disclaimer
References
49 Laser‐Based Navigation
49.1 Introduction
49.2 Laser‐Based Sensor Technology and Their Observables
49.3 Laser‐Based Navigation Approaches
49.3.1 Feature‐Based Laser Navigation
49.3.1.1 Geometric Feature Extraction
Algorithm: Split and Merge
49.3.1.2 Line and Planar‐Surface‐Based Navigation Mechanization
49.3.1.3 Feature‐Based Integrated Navigation
49.3.1.4 Feature‐Based SLAM
49.3.2 Featureless Laser‐Based Navigation
49.3.2.1 Point Cloud Matching
Algorithm: ICP
49.3.2.2 Featureless integrated Navigation
49.3.2.3 Laser‐Based Terrain Navigation Using Point Cloud Matching
49.3.2.4 Occupancy Grid
49.4 General Considerations
References
50 Image‐Aided Navigation – Concepts and Applications
50.1 Introduction
50.2 Imaging System Model. 50.2.1 What Information Is in an Image?
50.2.2 A Mathematical Camera Model
50.2.3 Camera Calibration
50.2.4 Fundamental Components of the Image‐Aided Navigation Problem
50.3 Selection of Interest Areas
50.3.1 Grid‐Based Features
50.3.2 Corner Features
50.3.3 Line Features
50.3.4 Robust Corner Features
50.3.5 USAN Features
50.4 Correspondence Search
50.5 Pose Estimation
50.5.1 Visual Odometry
50.5.2 SLAM and Bundle Adjustment
50.5.3 Absolute Positioning Using Features at Known Locations
50.5.3.1 Pose Estimation Summary
50.6 Monocular Image Navigation Issues
50.7 Application of Additional Information to the Image‐Aided Navigation Solution
50.7.1 Incorporating Additional Information to a Homogeneous Imaging Sensor
50.7.2 Stereoscopic Ranging
50.7.3 Stadiametric Ranging
50.7.4 Optical Ranging Techniques
50.7.5 Environmental Constraints
50.8 Non‐Homogeneous Sensor Fusion
50.8.1 Inertial Sensors
50.8.2 Odometry
50.8.3 GNSS Augmentation
50.9 Conclusion
References
51 Digital Photogrammetry
51.1 Introduction
51.1.1 History of Photogrammetry
51.1.2 Digital Photogrammetry
51.1.3 Photogrammetry and Navigation
51.2 Optical Imagery
51.2.1 Imaging Sensors
51.2.2 Image Properties
51.3 Basic Definitions. 51.3.1 Pinhole Camera Model
51.3.2 Coordinate Systems
51.3.3 Coordinate System Transformations
51.4 Fundamentals of Photogrammetry. 51.4.1 Interior Orientation Parameters
51.4.1.1 Linear Interior Orientation Parameters
51.4.1.2 Lens Distortion Parameters
51.4.2 Exterior Orientation
51.4.3 Stereo Photogrammetry
51.4.4 Computer Vision Versus Photogrammetry
51.5 Photogrammetric Processing Workflow
51.5.1 Camera Calibration
51.5.2 Exterior Orientation
51.5.2.1 Tie Point Generation
51.5.2.2 Bundle Adjustment
51.5.2.3 Error Model and Quality Control
51.5.3 Epipolar Resampling
51.5.4 Dense Reconstruction
51.5.5 Digital Elevation Model (DEM)
51.5.6 Orthorectification
51.6 Applications
51.6.1 Close‐Range Photogrammetry and Indoor Mapping
51.6.2 Aerial Photogrammetry
51.6.3 UAS and Mobile Mapping
51.6.4 Spaceborne Photogrammetry
51.7 Conclusion
Notations
References
52 Navigation Using Pulsars and Other Variable Celestial Sources
52.1 Navigation Concepts and Benefits
52.1.1 Background and Brief History of Research
52.1.2 Signal Processing Methods and Techniques
52.2 Variable Celestial Sources
52.2.1 Pulsars. 52.2.1.1 Periodic Sources
52.2.1.2 Emission Bands
52.2.2 Other Variable Stellar Sources for Navigation. 52.2.2.1 Aperiodic Sources
52.2.2.2 Gamma‐Ray Bursts
52.2.2.3 Additional Unique Celestial Sources
52.2.3 Variable Celestial Source Summary for Navigation
52.3 Applications for Spacecraft and Planetary Vehicles
52.3.1 Position and Velocity Determination
52.3.1.1 Absolute Solutions
52.3.1.2 Delta‐Correction Solutions
52.3.1.3 Relative Navigation
52.3.2 Attitude Determination
52.3.3 Time Correction and Synchronization
52.3.4 Pulsar Time Scale
52.3.5 Enhanced Communication Techniques
52.4 Current Technology Limitations and Future Developments
52.4.1 Improving Photon Detection and Timing
52.4.2 Future Detection Approaches
52.4.3 Ongoing and Future Demonstrations
Acknowledgments
Acronyms
References
53 Neuroscience of Navigation
53.1 Introduction
53.2 Spatial Foundations
53.3 Specialized Spatial Cells
53.3.1 Place Cells
53.3.2 Head Direction Cells
53.3.3 Grid Cells
53.3.4 Speed and Boundary Cells
53.3.5 Future Directions
53.4 Neural Systems and Navigation
53.4.1 Hippocampal versus Striatal Systems
53.4.2 Additional Brain Areas Involved in Navigation
53.4.2.1 Posterior Parietal Cortex
53.4.2.2 Posterior Parahippocampal Place Area
53.4.2.3 Entorhinal Cortex
53.4.2.4 Retrosplenial Cortex
53.4.2.5 Prefrontal Cortex
53.4.3 Brain Networks
53.4.4 Aging and Navigation
53.5 Future Directions. 53.5.1 Different Spatial Scales
53.5.2 Three‐Dimensional Navigation
53.6 Conclusion
References
54 Orientation and Navigation in the Animal World
54.1 What Information Do Animals Use to Orientate and Navigate?
Box 54.1 Genetic instructions and cultural transmission
54.1.1 Celestial Cues
54.1.2 The Sun
54.1.3 Polarized Light
Box 54.2 The polarized sky
54.2 Star Compass
54.2.1 Olfaction
54.2.2 The Geomagnetic Field
54.2.3 Mechanisms to Sense Magnetic Fields
54.3 Radical‐Pair‐Based Magnetoreceptor
54.3.1 Magnetite‐Based Magnetoreceptors
54.4 Conclusions and Perspectives
References
55 GNSS Applications in Surveying and Mobile Mapping
55.1 Introduction
55.2 Surveying and Mobile Mapping Sector’s Positioning Requirements
55.3 GNSS Applications in Land and Marine Surveying
55.3.1 Cadastral Surveying and Land Management
55.3.2 Construction Surveying
55.3.3 Mapping
55.3.4 Mine Surveying
55.3.5 Infrastructure Monitoring
55.3.6 Marine Surveying
55.4 GNSS for Mobile Mapping
55.5 Emerging Developments in GNSS Systems and Mapping Industry
55.5.1 Expansion of Augmented GNSS Coverage
55.5.2 Augmented Reality (AR) for Surveying Applications
55.5.3 Emerging MMS
55.5.4 Conclusions and Future Outlook
References
56 Precision Agriculture
56.1 Precision Agriculture
56.1.1 Accuracy and Repeatability Requirements
56.1.2 Automatic Steering
56.1.3 Soil Sampling
56.1.4 Variable Rate Application of Seeds and Chemicals
56.1.5 Section Control
56.1.6 Harvest Yield Mapping
56.1.7 Water Management
56.1.7.1 Land Leveling
56.1.7.2 VR Irrigation
56.1.8 Crop Scouting
56.1.9 Asset Tracking
56.2 GNSS Requirements for Agriculture
56.2.1 Receiver Random Clock Errors
56.2.2 Troposphere Effects
56.2.3 Multipath Signal Reception Errors
56.2.4 Reference Station Position Errors
56.2.5 Multiple GNSS Available
56.2.6 GNSS Correction Services
56.2.7 Plate Tectonic Effects on GNSS Correction Services
56.3 Conclusion
57 Wearables
57.1 Introduction
57.2 Origins of Wearables
57.3 The World of Wearables
57.3.1 Sport and Fitness
57.3.2 Smartwatches
57.3.3 Smart Clothing
57.3.4 Smart Jewelry
57.3.5 Health and Wellness
57.4 Wearable Device Architecture
57.4.1 Operating Systems
57.4.1.1 General‐Purpose Operating Systems (GPOSs)
57.4.1.2 Real‐Time Operating System
57.5 Sensors and Measurement
57.5.1 Position and Direction Sensors. 57.5.1.1 GNSS
57.5.1.1.1 Signal Acquisition
57.5.1.1.2 Antennas and Reception
57.5.1.1.3 Dead Reckoning
57.5.1.1.4 Map Matching
57.5.1.2 Gyroscope
57.5.1.3 Magnetometer
57.5.2 Movement and Acceleration (Inertial) Sensors. 57.5.2.1 Accelerometer
57.5.2.2 Pedometer
57.5.3 Biophysical Sensors. 57.5.3.1 Heart Rate Measurement
57.5.3.2 Calorie Measurement
57.5.3.3 Blood Pressure
57.5.3.4 Galvanic Skin Response (GSR)
57.5.3.5 Thermometer
57.5.3.6 Perspiration
57.5.4 Environmental Sensors. 57.5.4.1 Air Pressure
57.5.4.2 Temperature and Humidity Sensors
57.6 Power Management/Battery Monitoring
57.7 Screens
57.8 Video and Audio. 57.8.1 Video
57.8.2 Audio
57.9 Wireless Technology
57.9.1 Bluetooth Smart or BLE
57.9.2 ANT(+)
57.9.3 Near‐Field Communication (NFC)
57.9.4 Coexistence
57.9.5 Comparisons
57.10 Privacy and Security
57.11 The Future
57.11.1 Wearable Devices
57.11.2 Sensors
57.11.3 Indoor Navigation and Positioning
57.12 Summary
58 Navigation in Advanced Driver Assistance Systems and Automated Driving
58.1 Introduction
58.2 Useful GNSS Measurements for Vehicle Automation
58.3 Vehicle Modeling
58.4 Applications
58.4.1 GNSS Vehicle State Estimation
58.4.2 Vehicle Navigation
58.4.2.1 Vehicle Motion Constraints
58.4.2.2 Vehicle Dynamic Model
58.4.2.3 Onboard Sensors
58.4.2.4 GPS Integrity and Robustness Improvements for Ground Vehicles
58.4.3 Vehicle Following
58.4.3.1 Lateral Control
References
59 Train Control and Rail Traffic Management Systems
59.1 The Role of GNSS in Modern Train Control Systems
59.1.1 General Train Control
59.1.2 Implementation of GNSS Within the ERTMS/ETCS Framework
59.1.3 Integrity Considerations
59.2 Track‐Constrained PNT
59.3 GNSS and Odometer Fusion
59.4 Track‐Constrained Relative PVT Estimate
59.5 Multiple‐Track Discrimination
59.6 Track Detector Performance
References
60 Commercial Unmanned Aircraft Systems (UAS)
60.1 UAS Context. 60.1.1 Commercial Application Domains
60.1.2 Operational Environments and Regulations
60.1.3 UAS Traffic Management (UTM)
60.1.4 Summary of Emergent Challenges
60.2 Flight Guidance and Autonomy. 60.2.1 Guidance, Navigation, Command and Control
60.2.2 Autonomy
60.2.3 Motion Planning
60.3 Obstacle Avoidance: Environment. 60.3.1 Risk Assessment
60.3.2 Geo‐Fencing
60.3.3 Assured Containment
60.4 Obstacle Avoidance: Other Aerial Vehicles
60.4.1 From “See and Avoid” to “Sense and Avoid”
60.4.2 Well Clear Definitions
60.4.3 Collision Avoidance Systems for Cooperative Aircraft
60.4.4 Example SAA Implementations
60.4.5 Encounter with Noncooperative Intruders
60.5 Role of Navigation
60.5.1 Alternative sUAS Navigation – Using Laser Range Scanner
60.5.2 Alternative sUAS Navigation – Using Vision Sensors
60.5.3 Alternative sUAS Navigation – Using Signals‐of‐Opportunity
References
61 Navigation for Aviation
61.1 Introduction and Overview
61.2 Navigation for Aviation: Past and Present. 61.2.1 A Brief History
61.2.2 Today’s Airways
61.2.3 A Vision of the Future
61.3 Aviation Navigation for the 21st Century
61.4 Satellite Navigation
61.5 Terrestrial Radio Navigation Sources
61.5.1 DME and Tactical Navigation
61.5.2 Enhanced DME
61.5.3 Instrument Landing System
61.5.4 VHF Omnidirectional Ranging
61.5.5 NDB and ADF
61.6 Surveillance‐Based Navigation
61.6.1 Surveillance and Navigation
61.6.2 Automatic Dependent Surveillance Broadcast and Related Signals
61.6.3 ADS‐B Transmission for Navigation
61.7 Signals of Opportunity
61.8 Naturally Occurring Aviation Signal
61.8.1 Inertial Navigation System
61.8.2 Barometric altimeter
61.8.3 Magnetic Compass
61.9 Vision
61.10 Conclusions
References
Notes
62 Orbit Determination with GNSS
62.1 Introduction
62.2 Formulation of the Orbit Determination Problem
62.3 The First Step in Solving the POD Problem: Linearization
62.4 Types of Orbit Determination Approaches: Kinematic, Dynamics, and “In Between,” Also Known as Reduced Dynamics
62.5 The Critical Role of the Reference GNSS Orbit and Clock States
62.6 POD Solution Validation
62.7 LEOs, MEOs, and HEOs
62.8 Formation Flying and Relative Positioning
62.9 Elements of the Art. 62.9.1 Receiver Performance
62.9.2 Force Models (Including Attitude Models)
62.9.3 Relativistic Effects on Clocks
62.9.4 Measurement Models (Including Antenna and Media; Multipath, Single Frequency Versus Dual Frequency; Empirical Antenna Models)
62.9.5 Orbit Integration
62.9.6 Ambiguity Resolution
62.9.7 Tracking Data and Data Weight
62.10 Timing
62.11 Orbit Determination for Earth Science
62.12 Synergy with Other Data Types
62.13 Onboard Orbit Determination
62.14 Case Study: Jason‐3 Mission
63.3.4 Acknowledgments
References
63 Satellite Formation Flying and Rendezvous
63.1 Introduction to Relative Navigation
63.1.1 History and State of the Art
63.1.1.1 Rendezvous Missions
63.1.1.2 Formation‐Flying Technology Demonstration Missions
63.1.1.3 Formation‐Flying Science Missions
63.1.2 Potential and Future Applications
63.2 Relative Orbit Determination
63.2.1 State Representations and Dynamics Models
63.2.1.1 Dual‐Inertial State Representation
63.2.1.2 Linearized Relative Dynamics Using Spherical Coordinates
63.2.1.3 Linearized Relative Dynamics using Orbit Elements
63.2.2 State Predictability
63.2.3 Estimation
63.2.3.1 Editing
63.2.3.2 Underweighting
63.2.3.3 Factorization
63.2.3.4 Bias Modeling
63.3 Mission Results
63.3.1 Space Shuttle
63.3.2 PRISMA
63.3.3 TanDEM‐X
63.3.4 MMS
63.4 Conclusions
References
64 Navigation in the Arctic
64.1 Introduction
64.2 Ice Navigation
64.2.1 Charts and Surveying in Remote Areas
64.2.2 High‐Latitude Challenges for Satellite Navigation
64.2.3 Offshore Positioning
64.2.4 Ice Information, Routing, and Management
64.3 21st Century Ice Navigation
64.3.1 Ship‐Based Sensing
64.3.2 Situational Awareness
64.3.2.1 ESABALT Platform
64.3.3 Ice‐Aware Routing
64.4 GNSS Integrity in the Arctic
64.4.1 SBAS and ARAIM in the Arctic
64.4.2 Maritime and Aviation Requirements
64.4.3 Service Levels from SBAS and ARAIM
64.5 Conclusions
References
Glossary, Definitions, and Notation Conventions. Glossary
Algebraic Conventions
PVT Computation Variables and Notation
Variables
Signal Processing Variables and Notation
Orbital Variables and Notation
Signal Propagation Variables and Notation
Index. a
b
c
d
e
f
g
h
i
j
k
l
m
n
o
p
q
r
s
t
u
v
w
x
z
WILEY END USER LICENSE AGREEMENT
Отрывок из книги
IEEE Press 445 Hoes Lane Piscataway, NJ 08854
.....
Figure 36.14 Grid particle filter state estimate (after 100 observations). Note that the state estimate is almost completely unimodal and has converged to the correct integer ambiguity.
After 22 cycles, the density shows a reduced number of peaks (see Figure 36.13). This indicates that the filter is incorporating sensor observations and the statistical dynamics model to effectively eliminate a number of potential ambiguity possibilities.
.....