Organofluorine Chemistry
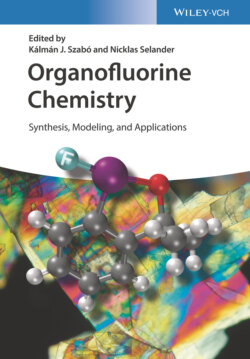
Реклама. ООО «ЛитРес», ИНН: 7719571260.
Оглавление
Группа авторов. Organofluorine Chemistry
Table of Contents
Pages
Organofluorine Chemistry. Synthesis, Modeling, and Applications
Preface
1 The Development of New Reagents and Reactions for Synthetic Organofluorine Chemistry by Understanding the Unique Fluorine Effects
1.1 Introduction
1.2 The Unique Fluorine Effects in Organic Reactions
1.2.1 Fluorine‐Enabled Stability of “CuCF3” in Water, and the Unusual Water‐Promoted Trifluoromethylation
1.2.2 Fluorine Enables β‐Fluoride Elimination of Organocopper Species
1.2.3 The “Negative Fluorine Effect” Facilitates the α‐Elimination of Fluorocarbanions to Generate Difluorocarbene Species
1.2.4 Tackling the β‐Fluoride Elimination of Trifluoromethoxide Anion via a Fluoride Ion‐Mediated Process
1.3 The Relationships Among Fluoroalkylation, Fluoroolefination, and Fluorination
1.3.1 From Fluoroalkylation to Fluoroolefination
1.3.2 From Fluoroolefination to Fluoroalkylation
1.3.3 From Fluoroalkylation to Fluorination
1.4 Conclusions
References
2 Perfluoroalkylation Using Perfluorocarboxylic Acids and Anhydrides
2.1 Introduction
2.2 Perfluoroalkylation with Perfluorocarboxylic Acids
2.2.1 Electrochemical Reactions
2.2.1.1 Reactions of Alkenes and Alkynes
2.2.1.2 Reaction of Aromatic Compounds
2.2.2 Reactions Using XeF2
2.2.3 Reactions Using Copper and Silver Salts
2.2.3.1 Using Copper Salts
2.2.3.2 Using Silver Salts
2.2.4 Photochemical Reactions
2.2.5 Other Methods. 2.2.5.1 Hydro‐Trifluoromethylation of Fullerene
2.2.5.2 Metal‐Free Aryldifluoromethylation Using S2O82−
2.3 Perfluoroalkylation with Perfluorocarboxylic Anhydride
2.3.1 Reactions Using Perfluorocarboxylic Anhydride/Urea·H2O2
2.3.2 Photocatalytic Reactions Using Perfluorocarboxylic Anhydride/Pyridine N‐oxide
2.4 Summary and Prospects
References
3 Chemistry of OCF3, SCF3, and SeCF3 Functional Groups
3.1 Introduction
3.2 CF3O Chemistry
3.2.1 De Novo Construction. 3.2.1.1 Trifluorination of Alcohol Derivatives
3.2.1.2 Fluorination of Difluorinated Compounds
3.2.2 Indirect Methods
3.2.2.1 O‐(Trifluoromethyl)dibenzofuranium Salts
3.2.2.2 Hypervalent Iodine Trifluoromethylation Reagents
3.2.2.3 CF3SiMe3
3.2.3 Direct Trifluoromethoxylation
3.2.3.1 Difluorophosgene and Derivatives
3.2.3.2 Trifluoromethyl Hypofluorite and Derivatives
3.2.3.3 Trifluoromethyl Triflate (TFMT)
3.2.3.4 Trifluoromethoxide Salts Derived from TFMT or Difluorophosgene
3.2.3.5 Trifluoromethyl Arylsulfonates (TFMSs)
3.2.3.6 Trifluoromethylbenzoate (TFBz)
3.2.3.7 2,4‐Dinitro(trifluoromethoxy)benzene (DNTFB)
3.2.3.8 (Triphenylphosphonio)difluoroacetate (PDFA)
3.2.3.9 N‐Trifluoromethoxylated Reagents
3.3 CF3S Chemistry
3.3.1 Indirect Methods
3.3.2 Direct Trifluoromethylthiolation
3.3.2.1 CF3SAg, CF3SCu, CF3SNR4
3.3.2.2 Trifluoromethanesulfenamides
3.3.2.3 N‐Trifluoromethylthiophthalimide
3.3.2.4 N‐Trifluoromethylthiosaccharin
3.3.2.5 N‐Trifluoromethylthiobis(phenylsulfonyl)amide
3.4 CF3Se Chemistry. 3.4.1 Introduction
3.4.2 Indirect Synthesis of CF3Se Moiety
3.4.2.1 Ruppert–Prakash Reagent (CF3SiMe3)
3.4.2.2 Fluoroform (HCF3)
3.4.2.3 Other Reagents Involved in CF3− Anion Generation
3.4.2.4 Sodium Trifluoromethylsulfinate (CF3SO2Na)
3.4.3 Direct Introduction of the CF3Se Moiety
3.4.3.1 Trifluoromethyl Selenocopper DMF Complex
3.4.3.2 Trifluoromethyl Selenocopper Bipyridine Complex: [bpyCuSeCF3]2
3.4.3.2.1 C(sp3)—Se Bond Formation
3.4.3.2.2 C(sp2)—Se Bond Formation
3.4.3.2.3 C(sp)—Se Bond Formation
3.4.3.3 Tetramethylammonium Trifluoromethylselenolate [(NMe4)(SeCF3)]
3.4.3.4 In Situ Generation of CF3Se− Anion from Elemental Selenium
3.4.3.5 Trifluoromethylselenyl Chloride (CF3SeCl)
3.4.3.6 Benzyltrifluoromethylselenide (CF3SeBn)
3.4.3.7 Trifluoromethylselenotoluenesulfonate (CF3SeTs)
3.4.3.7.1 CF3SeTs as Electrophilic Reagent
3.4.3.7.2 CF3SeTs as Nucleophilic Reagent
3.4.3.7.3 CF3SeTs in Radical Trifluoromethylselenolation
3.4.3.8 Benzylthiazolium Salt BT‐SeCF3
3.5 Summary and Conclusions
References
4 Introduction of Trifluoromethylthio Group into Organic Molecules
4.1 Introduction
4.2 Nucleophilic Trifluoromethylthiolation. 4.2.1 Preparation of Nucleophilic Trifluoromethylthiolating Reagent. 4.2.1.1 Preparation of Hg(SCF3)2, AgSCF3, and CuSCF3
4.2.1.2 Preparation of MSCF3 (M = K, Cs, Me4N, and S(NMe2)3)
4.2.1.3 Preparation of Stable Trifluoromethylthiolated Copper(I) Complexes
4.2.2 Formation of C(sp2)‐SCF3 by Nucleophilic Trifluoromethylthiolating Reagents. 4.2.2.1 Reaction of CuSCF3 with Aryl Halides
4.2.2.2 Sandmeyer‐Type Trifluoromethylthiolation
4.2.2.3 Transition Metal‐Catalyzed Trifluoromethylthiolation
4.2.2.4 Oxidative Trifluoromethylthiolation
4.2.2.5 Transition Metal‐Catalyzed Trifluoromethylthiolation of Arenes via C–H Activation
4.2.2.6 Miscellaneous Methods for the Formation or Aryl Trifluoromethylthioethers via Nucleophilic Trifluoromethylthiolating Reagents
4.2.3 Formation of C(sp3)‐SCF3 by Nucleophilic Trifluoromethylthiolating Reagents. 4.2.3.1 Reaction of CuSCF3 with Activated Alkylated Halides
4.2.3.2 Reaction of MSCF3 with Unactivated Alkyl Halides
4.2.3.3 Nucleophilic Dehydroxytrifluoromethylthiolation of Alcohols
4.2.3.4 Nucleophilic Trifluoromethylthiolation of Alcohol Derivatives
4.2.3.5 Nucleophilic Trifluoromethylthiolation of α‐Diazoesters
4.2.3.6 Formation or Alkyl Trifluoromethylthioethers via In Situ Generated Nucleophilic Trifluoromethylthiolating Reagent
4.2.3.7 Formation of Alkyl Trifluoromethylthioethers via C—H Bond Trifluoromethylthiolation
4.3 Electrophilic Trifluoromethylthiolating Reagents
4.3.1 CF3SCl
4.3.2 CF3SSCF3
4.3.3 Haas Reagent
4.3.4 Munavalli Reagent
4.3.5 Billard Reagent
4.3.6 Shen Reagent
4.3.7 Shen Reagent‐II
4.3.8 Optically Active Pure Trifluoromethylthiolation Reagents
4.3.9 Lu–Shen Reagent
4.3.10 α‐Cumyl Bromodifluoromethanesulfenate
4.3.11 Shibata Reagent
4.3.12 In Situ‐Generated Electrophilic Trifluoromethylthiolating Reagents
4.3.12.1 AgSCF3 + TCCA
4.3.12.2 AgSCF3 + NCS
4.3.12.3 Langlois Reagent (CF3SO2Na) with Phosphorus Reductants
4.3.12.4 Use of CF3SO2Cl with Phosphorus Reductants
4.3.12.5 Reagent Based on CF3SOCl and Phosphorus Reductants
4.4 Radical Trifluoromethylthiolation
4.4.1 Trifluoromethylthiolation by AgSCF3/S2O82−
4.4.2 Electrophilic Reagents Involved in Radical Trifluoromethylthiolation
4.4.3 Visible Light‐Promoted Trifluoromethylthiolation by Using Electrophilic Reagents
4.5 Summary and Prospect
References
5 Bifunctionalization‐Based Catalytic Fluorination and Trifluoromethylation
5.1 Introduction
5.2 Palladium‐Catalyzed Fluorination, Trifluoromethylation, and Trifluoromethoxylation of Alkenes
5.2.1 Palladium‐Catalyzed Fluorination of Alkenes
5.2.2 Palladium‐Catalyzed Trifluoromethylation of Alkenes
5.2.3 Palladium‐Catalyzed Trifluoromethoxylation of Alkenes
5.3 Copper‐Catalyzed Trifluoromethylative Functionalization of Alkenes
5.3.1 Copper‐Catalyzed Trifluoromethylamination of Alkenes
5.3.2 Copper‐Catalyzed Trifluoromethyloxygenation of Alkenes
5.3.3 Copper‐Catalyzed Trifluoromethylcarbonation of Alkenes
5.3.4 Enantioselective Copper‐Catalyzed Trifluoromethylation of Alkenes
5.4 Summary and Conclusions
References
6 Fluorination, Trifluoromethylation, and Trifluoromethylthiolation of Alkenes, Cyclopropanes, and Diazo Compounds
6.1 Introduction
6.2 Fluorination of Alkenes, Cyclopropanes, and Diazocarbonyl Compounds
6.2.1 Application of Fluoro‐Benziodoxole for Fluorination of Alkenes
6.2.1.1 Geminal Difluorination of Styrene Derivatives
6.2.1.2 Iodofluorination of Alkenes
6.2.1.3 Fluorocyclization with C—N, C—O, and C—C Bond Formation
6.2.2 Fluorinative Cyclopropane Opening
6.2.3 Fluorine‐18 Labeling with Fluorobenziodoxole
6.3 Fluorination‐Based Bifunctionalization of Diazocarbonyl Compounds
6.3.1 Rhodium‐Catalyzed Geminal Oxyfluorination Reactions
6.3.2 [18F]Fluorobenziodoxole for Synthesis of α‐Fluoro Ethers
6.4 Trifluoromethylation of Alkenes, Alkynes, and Diazocarbonyl Compounds with the Togni Reagent
6.4.1 Bifunctionalization of C—C Multiple Bonds
6.4.1.1 Oxytrifluoromethylation of Alkenes and Alkynes
6.4.1.2 Cyanotrifluoromethylation of Styrenes
6.4.1.3 C–H Trifluoromethylation of Benzoquinone Derivatives
6.4.2 Geminal Oxytrifluoromethylation of Diazocarbonyl Compounds
6.5 Bifunctionalization‐Based Trifluoromethylthiolation of Diazocarbonyl Compounds
6.5.1 Multicomponent Approach for Geminal Oxy‐Trifluormethylthiolation
6.5.2 Simultaneous Formation of C—C and C—SCF3 Bonds via Hooz‐Type Reaction
6.6 Summary
References
7 Photoredox Catalysis in Fluorination and Trifluoromethylation Reactions
7.1 Introduction
7.2 Fluorination
7.2.1 Fluorination Through Direct HAT Process by Excited Photocatalyst
7.2.2 Fluorination Through Photoredox Processes
7.3 Trifluoromethylation
7.3.1 Trifluoromethylation of Aromatic Compounds
7.3.2 Trifluoromethylative Substitution of Alkyl Bromides
7.4 Summary and Outlook
References
8 Asymmetric Fluorination Reactions
8.1 Introduction
8.2 Electrophilic Fluorination
8.2.1 Stoichiometric Asymmetric Fluorination. 8.2.1.1 Chiral Auxiliary
8.2.1.2 Chiral Reagents
8.2.2 Catalytic Electrophilic Fluorination. 8.2.2.1 Organocatalytic Fluorination
8.2.2.1.1 Tertiary Amine Catalysis
8.2.2.1.2 Chiral Cation Phase Transfer
8.2.2.1.3 Enamine Catalysis
8.2.2.1.4 Chiral Lewis Base Catalysis
8.2.2.1.5 Chiral Anion Phase Transfer
8.2.2.2 Transition Metal‐Catalyzed Fluorinations
8.2.2.2.1 Fluorination of Metal Enolates or Equivalent
Ti–Taddol Complexes
BINAP‐ and SEGPHOS‐Based Ligands
Oxazoline‐Based Ligands
Miscellaneous Metal‐Catalyzed Reactions
8.2.2.2.2 Fluorination of Metal–Carbon Bonds Without Enolates
8.3 Nucleophilic Fluorination
8.3.1 Metal‐Catalyzed Nucleophilic Fluorination. 8.3.1.1 Ring Opening of Strained Ring Systems
8.3.1.2 Allylic Functionalization
8.3.2 Organocatalytic Nucleophilic Fluorination
8.4 Summary and Conclusions
References
9 The Self‐Disproportionation of Enantiomers (SDE): Fluorine as an SDE‐Phoric Substituent
9.1 Introduction
9.2 General Concepts and the Role of Fluorine in the Manifestation of the SDE
9.3 The SDE Phenomenon. 9.3.1 SDE via Distillation
9.3.2 SDE via Sublimation
9.3.3 SDE via Chromatography
9.3.3.1 SDEvC for Compounds Containing a –CF3 Moiety
9.3.3.2 SDEvC for Compounds Containing a Cq–F1/2 Moiety
9.3.3.3 SDEvC for Compounds Containing a –COCF3 Moiety
9.4 The SIDA Phenomenon
9.5 Conclusions and Recommendations
References
10 DFT Modeling of Catalytic Fluorination Reactions: Mechanisms, Reactivities, and Selectivities
10.1 Introduction
10.2 DFT Modeling of Transition Metal‐Catalyzed Fluorination Reactions. 10.2.1 Ti‐Catalyzed Fluorination Reaction
10.2.2 Mn‐Catalyzed Fluorination Reactions
10.2.3 Fe‐Catalyzed Fluorination Reactions
10.2.4 Rh‐Catalyzed Fluorination Reactions
10.2.5 Ir‐Catalyzed Fluorination Reactions
10.2.6 Pd‐Catalyzed Fluorination Reactions. 10.2.6.1 Pd‐Catalyzed Nucleophilic Fluorination
10.2.6.2 Pd‐Catalyzed Electrophilic Fluorination
10.2.7 Cu‐Catalyzed Fluorination Reactions. 10.2.7.1 Cu‐Catalyzed Nucleophilic Fluorination
10.2.7.2 Cu‐Mediated Radical Fluorination
10.2.8 Ag‐Catalyzed Fluorination Reactions
10.2.9 Zn‐Catalyzed Fluorination Reactions
10.3 DFT Modeling of Organocatalytic Fluorination Reactions. 10.3.1 Fluorination Reactions Catalyzed by Chiral Amines. 10.3.1.1 Chiral Secondary Amines‐Catalyzed Fluorination Reactions
10.3.1.2 Chiral Primary Amines‐Catalyzed Fluorination Reactions
10.3.2 Tridentate Bis‐Urea Catalyzed Fluorination Reactions
10.3.3 Hypervalent Iodine‐Catalyzed Fluorination Reactions
10.3.4 N‐Heterocyclic Carbene‐Catalyzed Fluorination Reactions
10.4 DFT Modeling of Enzymatic Fluorination Reaction
10.5 Conclusions
Acknowledgments
References
11 Current Trends in the Design of Fluorine‐Containing Agrochemicals
11.1 Introduction
11.2 Role of Fluorine in the Design of Modern Agrochemicals
11.3 Fluorinated Modern Agrochemicals
11.3.1 Herbicides Containing Fluorine. 11.3.1.1 Acetohydroxyacid Synthase/Acetolactate Synthase Inhibitors
11.3.1.2 Protoporphyrinogen Oxidase Inhibitors
11.3.1.3 Cellulose Biosynthesis Inhibitors
11.3.1.4 Very Long‐Chain Fatty Acid Synthesis Inhibitors
11.3.1.5 Auxin Herbicides
11.3.1.6 Hydroxyphenylpyruvate Dioxygenase Inhibitors
11.3.1.7 Selected Fluorine‐Containing Herbicide Development Candidates
11.3.2 Fungicides Containing Fluorine. 11.3.2.1 Fungicidal Succinate Dehydrogenase Inhibitors
11.3.2.2 Complex III Inhibitors
11.3.2.3 Sterolbiosynthesis (Sterol‐C14‐Demethylase) Inhibitors
11.3.2.4 Polyketide Synthase Inhibitors
11.3.2.5 Oxysterol‐Binding Protein Inhibitors
11.3.2.6 Selected Fluorine‐Containing Fungicide Development Candidates
11.3.3 Insecticides Containing Fluorine. 11.3.3.1 Nicotinic Acetylcholine Receptor Competitive Modulators
11.3.3.1.1 Sulfoximines
11.3.3.1.2 Butenolides
11.3.3.1.3 Mesoionics
11.3.3.2 Ryanodine Receptor (RyR) Modulators
11.3.3.3 GABA‐Gated CI‐Channel Allosteric Modulators
11.3.3.4 Selected Fluorine‐Containing Insecticide Development Candidates
11.3.4 Acaricides Containing Fluorine. 11.3.4.1 Mitochondrial Complex II Electron Transport Inhibitors
11.3.4.2 Selected Fluorine‐Containing Acaricide Development Candidates
11.3.5 Nematicides Containing Fluorine. 11.3.5.1 Nematicides with Unknown Biochemical MoA
11.3.5.2 Nematicidal Succinate Dehydrogenase Inhibitors
11.3.5.3 Selected Fluorine‐Containing Nematicide Development Candidates
11.4 Summary and Prospects
References
12 Precision Radiochemistry for Fluorine‐18 Labeling of PET Tracers
12.1 Introduction
12.2 Electrophilic 18F‐Fluorination with [18F]F2 and [18F]F2‐Derived Reagents
12.3 Nucleophilic Aliphatic 18F‐Fluorination. 12.3.1 Transition Metal‐Free Nucleophilic Aliphatic Substitution with [18F]Fluoride
12.3.2 Transition Metal‐Mediated Aliphatic 18F‐Fluorination
12.4 Nucleophilic Aromatic 18F‐Fluorination with [18F]Fluoride. 12.4.1 Transition Metal‐Free Nucleophilic Aromatic 18F‐Fluorination with [18F]Fluoride
12.4.2 Transition Metal‐Mediated Aromatic 18F‐Fluorination
12.5 18F‐Labeling of Multifluoromethyl Motifs with [18F]Fluoride
12.6 Summary and Conclusions
References
Index
WILEY END USER LICENSE AGREEMENT
Отрывок из книги
Edited by Kálmán J. Szabó and Nicklas Selander
As mentioned above, all useful organofluorine compounds are human made. Therefore, there is an enormous pressure on synthetic organic chemists to produce a wide variety of new fluorinated compounds. This book is intended to cover the most important state‐of‐the‐art synthetic methods and addresses current challenges within the field. At the same time, it gives in‐depth analysis of the underlying chemical processes controlling the formation of new bonds between carbon and fluorine or fluorinated functional groups. A further important aspect is the application of organofluorine compounds in various areas of medicinal and agrochemistry.
.....
Except for 2‐PySO2CF2H, many other fluorinated heteroaryl sulfones have been extensively investigated. As we can see from Scheme 1.11c, although BTSO2CF2H was found to be an inefficient fluoroolefination reagent, its use for radical fluoroalkylation is a great success. In 2015, we developed a novel method for the preparation of sodium fluoroalkanesulfinates by the reduction of the corresponding benzo[d]thiazol‐2‐yl sulfones (BTSO2Rf) [9]. This method enables a highly efficient and rapid large‐scale synthesis of sodium di‐ and monofluoromethanesulfinates (Scheme 1.18a). Synthetic application of these sulfinates in radical fluoroalkylation is exemplified by the silver‐catalyzed cascade fluoroalkylation/aryl migration/SO2 extrusion of conjugated N‐arylsulfonylated amides (Scheme 1.18b).
The versatility of heteroaryl sulfones as fluoroalkyl radical precursors may be best illustrated by the readily tunable reactivity of heteroaryl sulfones that can generate a wide range of fluoroalkyl radicals such as monofluoromethyl, difluoromethyl, 1, 1‐difluoroethyl, phenyldifluoromethyl, benzoyldifluoromethyl, and trifluoromethyl radicals via visible light photoredox catalysis under mild conditions [7d]. The synthetic application of heteroaryl sulfones in radical fluoroalkylation of isocyanides was demonstrated to highlight the versatility of heteroaryl sulfones as fluoroalkyl radical precursors (Scheme 1.19a). The most prominent feature using heteroaryl sulfones as fluoroalkyl radical precursors is that they are reactivity‐tunable. By slightly changing the heteroaryl rings, the redox potential of fluorinated heteroaryl sulfones can be varied, ensuring the efficient generation of various fluoroalkyl radicals (Scheme 1.19b).
.....