Heterogeneous Catalysts
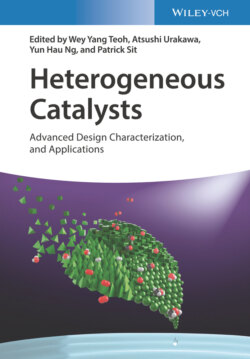
Реклама. ООО «ЛитРес», ИНН: 7719571260.
Оглавление
Группа авторов. Heterogeneous Catalysts
Table of Contents
List of Tables
List of Illustrations
Guide
Pages
Heterogeneous Catalysts. Advanced Design, Characterization and Applications
Heterogeneous Catalysts. Advanced Design, Characterization and Applications
Preface
1 Evolution of Catalysts Design and Synthesis: From Bulk Metal Catalysts to Fine Wires and Gauzes, and that to Nanoparticle Deposits, Metal Clusters, and Single Atoms
1.1 The Cradle of Modern Heterogeneous Catalysts
1.2 The Game Changer: High‐Pressure Catalytic Reactions
1.3 Catalytic Cracking and Porous Catalysts
1.4 Miniaturization of Metal Catalysts: From Supported Catalysts to Single‐Atom Sites
1.5 Perspectives and Opportunities
References
2 Facets Engineering on Catalysts
2.1 Introduction
2.2 Mechanisms of Facets Engineering
2.3 Anisotropic Properties of Crystal Facets
2.3.1 Anisotropic Adsorption
2.3.2 Surface Electronic Structure
2.3.3 Surface Electric Field
2.4 Effects of Facets Engineering
2.4.1 Optical Properties
2.4.2 Activity and Selectivity
2.5 Outlook
References
3 Electrochemical Synthesis of Nanostructured Catalytic Thin Films
3.1 Introduction
3.2 Principle of Electrochemical Method in Fabricating Thin Film
3.2.1 Anodization
3.2.1.1 Pulse or Step Anodization
3.2.2 Cathodic Electrodeposition
3.2.2.1 Pulse Electrodeposition
3.2.3 Electrophoretic Deposition
3.2.4 Combinatory Methods Involving Electrochemical Process
3.2.4.1 Combined Electrophoretic Deposition–Anodization (CEPDA) Approach
3.3 Conclusions and Perspective
References
4 Synthesis and Design of Carbon‐Supported Highly Dispersed Metal Catalysts
4.1 Introduction
4.2 Preparation of Catalysts on New Carbon Supports
4.2.1 Catalyst on Graphene Oxide
4.2.2 Catalyst on Graphene
4.2.2.1 Graphene or rGO as Starting Material
4.2.2.2 Graphene Oxide as Precursor of Graphene‐Supported Catalyst
4.2.2.3 Graphene Derivatives: Doped Graphene and Synthetic Derivatives
4.2.3 Catalyst on Nanodiamonds and Onion‐Like Carbon
4.2.4 SACs on Carbon Nitrides and Covalent Triazine Frameworks
4.2.5 Catalyst on Carbon Material from Hydrothermal Carbonization of Biomolecules
4.3 Emerging Techniques for Carbon‐Based Catalyst Synthesis
4.3.1 Deposition of Colloidal Nanoparticles
4.3.2 Single‐Metal Atom Deposition by Wet Chemistry
4.3.3 Immobilization of Metal Clusters and SACs by Organometallic Approach
4.3.4 Chemical Vapor Deposition Techniques on Carbon Supports
4.3.5 Simultaneous Formation of Metallic Catalyst and Porous Carbon Support by Pyrolysis
4.3.6 Dry Mechanical Methods
4.3.7 Electrodeposition
4.3.8 Photodeposition
4.4 Conclusions and Outlook
References
5 Metal Cluster‐Based Catalysts
5.1 Introduction
5.2 Catalysts Made by Deposition of Clusters from the Gas Phase Under Ultrahigh Vacuum
5.3 Chemically Synthesized Metal Clusters
5.4 Catalysis Using the Chemically Synthesized Metal Clusters
5.5 Conclusion
References
6 Single‐Atom Heterogeneous Catalysts
6.1 Introduction
6.2 Concept and Advantages of SACs. 6.2.1 Concept of SACs
6.2.2 Advantages of SACs. 6.2.2.1 Maximum Atom Efficiency
6.2.2.2 Unique Catalytic Properties
6.2.2.3 Identification of Catalytically Active Sites
6.2.2.4 Establishment of Intrinsic Reaction Mechanisms
6.3 Synthesis of SACs
6.3.1 Physical Methods
6.3.2 Chemical Methods
6.3.2.1 Bottom‐Up Synthetic Methods
6.3.2.2 Top‐Down Synthetic Methods
6.4 Challenges and Perspective
References
7 Synthesis Strategies for Hierarchical Zeolites
7.1 Introduction
7.2 Hierarchical Zeolites
7.2.1 Increased Intracrystalline Diffusion
7.2.2 Reduced Steric Limitation
7.2.3 Changed Product Selectivity
7.2.4 Decreased Coke Formation
7.3 Modern Strategies for the Synthesis of Hierarchical Zeolites
7.3.1 Hard Templates
7.3.1.1 Confined‐Space Method
7.3.1.2 Carbon Nanotubes and Nanofibers
7.3.1.3 Ordered Mesoporous Carbons
7.3.2 Soft Templates
7.3.2.1 Templating with Surfactants
7.3.2.2 Silanization Templating Methods
7.3.3 Dealumination
7.3.4 Desilication
7.4 Conclusion
References
8 Design of Molecular Heterogeneous Catalysts with Metal–Organic Frameworks
8.1 Secondary Building Units (SBUs) and Isoreticular MOFs
8.2 The Tools to Build Molecular Active Sites: Reticular Chemistry and Beyond
8.2.1 Pre‐synthetic Methodologies
8.2.2 Post‐synthetic Methodologies
8.2.2.1 Post‐synthetic Modification (PSM)
8.2.2.2 Post‐synthetic Exchange (PSE)
8.3 MOFs in Catalysis
8.3.1 The Difference Between MOFs and Standard Heterogeneous and Homogeneous Catalysts
8.4 Conclusion: Where to Go from Here
References
9 Hierarchical and Anisotropic Nanostructured Catalysts
9.1 Introduction
9.2 Top‐Down vs. Bottom‐Up Approaches
9.3 Shape Anisotropy and Nanostructured Assemblies
9.4 Janus Nanostructures
9.5 Hierarchical Porous Catalysts
9.6 Functionalization of Porous/Anisotropic Substrates
9.7 Perspective
References
10 Flame Synthesis of Simple and Multielemental Oxide Catalysts
10.1 From Natural Aerosols Formation to Engineered Nanoparticles
10.2 Flame Aerosol Synthesis and Reactors
10.3 Simple Metal Oxide‐Based Catalysts
10.4 Multielemental Oxide‐Based Catalysts
10.4.1 Solid Solution Metal Oxide Catalysts
10.4.2 Composite Metal Oxide Catalysts
10.4.3 Complex Metal Oxide Catalysts
10.5 Perspective and Outlook
References
11 Band Engineering of Semiconductors Toward Visible‐Light‐Responsive Photocatalysts
11.1 Basis of Photocatalyst Materials
11.2 Photocatalyst Material Groups. 11.2.1 Variety of Photocatalyst Materials
11.2.2 Main Constituent Metal Elements in Photocatalyst Materials
11.3 Design of Band Structures of Photocatalyst Materials
11.3.1 Doped Photocatalysts
11.3.2 Valence‐Band‐Controlled Photocatalysts
11.3.3 Solid Solution Photocatalysts
11.4 Preparation of Photocatalysts
11.4.1 Solid‐State Reaction Method
11.4.2 Flux Method
11.4.3 Hydrothermal Synthesis Method/Solvothermal Synthesis Method
11.4.4 Polymerized (Polymerizable) Complex Method
11.4.5 Precipitation Method
11.4.6 Loading of Cocatalysts
References
12 Toward Precise Understanding of Catalytic Events and Materials Under Working Conditions
References
13 Pressure Gaps in Heterogeneous Catalysis
13.1 Introduction
13.2 High‐Pressure Studies of Catalysts
13.3 Adsorption on Solid Surfaces at Low and High Pressures. 13.3.1 Kinetically Restricted Adsorbate Structures
Box 13.1 CO Adsorption Metal Surfaces. CO Adsorption on Pt(111)
CO Adsorption on Au Nanoparticles on CeO2
13.3.2 Thermodynamically Driven Reactions on Solid Surfaces
Box 13.2 CO Oxidation on Pt(110)
Box 13.3 Adsorption on Metal Oxides. CO Adsorption on TiO2(110)
HCOOH Adsorption on TiO2
13.3.3 Reactions on Supported Nanoparticle Catalysts
13.4 Conclusions and Outlook
Acknowledgments
References
14 In Situ Transmission Electron Microscopy Observation of Gas/Solid and Liquid/Solid Interfaces
14.1 Introduction
14.2 Observation in Gas and Liquid Phases
14.2.1 Window‐Type System
14.2.2 Differential Pumping‐Type System
14.2.3 Other Systems
14.3 Applications and Outlook
References
15 Tomography in Catalyst Design
15.1 Introduction
15.2 Imaging with X‐Rays
15.3 Conventional Absorption CT to Study Catalytic Materials
15.4 X‐Ray Diffraction Computed Tomography (XRD‐CT)
15.5 Pair Distribution Function CT
15.6 Multimodal XANES‐CT, XRD‐CT, and XRF‐CT
15.7 Atom Probe Tomography
15.8 Ptychographic X‐Ray CT
15.9 Conclusions
References
16 Resolving Catalyst Performance at Nanoscale via Fluorescence Microscopy
16.1 Fluorescence Microscopy as Catalyst Characterization Tool
16.2 Basics of Fluorescence and Fluorescence Microscopy
16.3 Strategies to Resolve Catalytic Processes in a Fluorescence Microscope
16.4 Wide‐Field and Confocal Fluorescence Microscopy
16.5 Super‐resolution Fluorescence Microscopy
16.6 What Can We Learn About Catalysts from (Super‐resolution) Fluorescence Microscopy: Case Studies
16.7 Conclusions and Outlook
References
17 In Situ Electron Paramagnetic Resonance Spectroscopy in Catalysis
17.1 Introduction
17.2 Basic Principles of Electron Paramagnetic Resonance (EPR)
17.3 Experimental Methods and Setup for In Situ cw‐EPR
17.4 Applications of In Situ EPR Spectroscopy
17.4.1 Cu‐Zeolite Systems
17.4.2 Radicals and Radical Ions
17.5 Conclusions
References
18 Toward Operando Infrared Spectroscopy of Heterogeneous Catalysts
18.1 Brief Theory on Infrared Spectroscopy
18.2 Different Modes of IR Measurements
18.3 Measuring the “Background”
18.4 Using Probe Molecules to Identify Heterogeneous Sites
18.5 IR Measurements Under Operando Conditions
18.6 Case Studies of Operando IR Spectroscopy. 18.6.1 Selective Catalytic Reduction of NO by NH3 Measured Using Operando Transmission IR
18.6.2 Methanation of CO2 Measured Using Operando DRIFTS
18.6.3 Selective Oxidation of Alcohols Measured Using Operando ATR‐IR
18.7 Perspective and Outlook
References
19 Operando X‐Ray Spectroscopies on Catalysts in Action
19.1 Fundamentals of X‐Ray Spectroscopy
19.2 X‐Ray Absorption Spectroscopy Methods
19.3 High‐Energy‐Resolution (Resonant) X‐Ray Emission Spectroscopy
19.4 In Situ and Operando Cells
19.5 Application of Time‐Resolved Methods
19.6 Limitations and Challenges
19.7 Concluding Remarks
References
20 Methodologies to Hunt Active Sites and Active Species
20.1 Introduction
20.2 Modulation Excitation Technique
20.3 Steady‐State Isotopic Transient Kinetic Analysis (SSITKA)
20.4 Multivariate Analysis
20.5 Outlook
References
21 Ultrafast Spectroscopic Techniques in Photocatalysis
21.1 Transient Absorption Spectroscopy. 21.1.1 Introduction
21.1.2 Conventional Heterogeneous Photocatalyst
21.1.3 Dye‐Sensitized Heterogeneous Photocatalyst
21.2 Time‐Resolved Photoluminescence. 21.2.1 Introduction
21.2.2 Applications of TRPL in Heterogeneous Catalysis
21.3 Time‐Resolved Microwave Conductivity. 21.3.1 Introduction
21.3.2 Applications of TRMC in Heterogeneous Catalysis
References
22 Quantum Approaches to Predicting Molecular Reactions on Catalytic Surfaces
22.1 Heterogeneous Catalysis and Computer Simulations
22.2 Theory of Quantum Mechanics
22.3 Quantum Mechanical Techniques in the Study of Heterogeneous Catalysis
References
23 Density Functional Theory in Heterogeneous Catalysis
23.1 Introduction
23.2 Basics of Density Functional Theory Calculations. 23.2.1 Born–Oppenheimer Approximation
23.2.2 The Hohenberg–Kohn Theorems and the Kohn–Sham Approach
23.2.3 Basis Sets
23.2.4 Forces on the Ions
23.3 The Search for Better Energy Functionals. 23.3.1 Energy Functional Development
23.3.2 Other Corrections and Approaches
23.4 DFT Applications in Heterogeneous Catalysis
23.5 Conclusions and Perspective
References
24 Ab Initio Molecular Dynamics in Heterogeneous Catalysis
24.1 Introduction
24.2 Basic Algorithm of Molecular Dynamics
24.2.1 Verlet Algorithm
24.2.2 Velocity Verlet Algorithm
24.2.3 Conserved Quantity
24.3 Molecular Dynamics in Canonical Ensembles
24.4 Transition State Theory
24.5 Free Energy Calculations
24.5.1 Thermodynamic Integration and Constrained MD
24.5.2 Umbrella Sampling
24.5.3 Metadynamics
24.6 Accelerating MD Simulations by Neural Network
24.7 Examples for MD Simulations
24.8 Conclusions
References
Chapter 25 First Principles Simulations of Electrified Interfaces in Electrochemistry
25.1 Toward Stable and High‐Performance Electrocatalysts
25.2 A Brief Thermodynamic Detour
25.2.1 The Fundamental Relation
25.2.2 Alternative Forms of the Fundamental Relation
25.3 Statistical Mechanics
25.3.1 Preliminaries
25.3.2 The Electrochemical Canonical Ensemble
25.3.3 The Electrochemical Grand Canonical Ensemble
25.3.4 Computational Methods
25.4 The Quantum‐Continuum Approach. 25.4.1 Overview
25.4.2 Electric Double Layer (EDL) Models
25.4.3 Example: Silver Monolayer Stripping on Au(100)
Acknowledgments
References
Notes
Chapter 26 Time‐Dependent Density Functional Theory for Excited‐State Calculations
26.1 Introduction
26.2 Theoretical Foundation of TDDFT
26.3 Linear Response Theory
26.4 Real‐Time TDDFT
26.5 Nonadiabatic Mixed Quantum/Classical Dynamics
References
27 The Method for Excited States Calculations
27.1 Introduction
27.2 Excitations in Many‐Electron Systems
27.3 Green's Functions
27.4 Many‐Body Perturbation Theory
27.5 in Practice
27.6 The Bethe–Salpeter Equation
27.7 BSE in Practice
27.8 Conclusions and Perspectives
References
28 High‐Throughput Computational Design of Novel Catalytic Materials
28.1 Introduction
28.2 The Framework of Computational Catalyst Design. 28.2.1 Elementary Reactions and Material Selection
28.2.2 The Scaling Relation and the Reaction Energy
28.2.3 The BEP Relation and the Activation Barrier
28.2.4 The Activity Volcano Curve
28.2.5 Explicit Kinetic Simulations Based on DFT Calculations
28.2.6 Data Mining and Machine Learning in Catalyst Design
28.3 Examples for Rational Catalyst Design
28.3.1 Synthesis of Higher Alcohols from Syngas on Alloys
28.3.2 HT Screening for Hydrogen Evolution Reactions (HERs)
28.3.3 Rational Design for CO Oxidation on Multicomponent Alloy Surfaces
28.3.4 Adsorbate–Adsorbate Interactions for CO Methanation
28.3.5 RhAu Alloy Nanoparticles for NO Decomposition by Machine Learning
28.4 Summary and Prospects of HT Catalytic Material Design
References
29 Embracing the Energy and Environmental Challenges of the Twenty‐First Century Through Heterogeneous Catalysis
References
30 Electrochemical Water Splitting
30.1 Fundamentals of Electrochemical Water Splitting
30.2 Technological and Practical Considerations
30.2.1 Liquid Electrolyte Water Electrolysis
30.2.1.1 Overall Water Electrolysis (OWE)
30.2.1.2 Doubled Water Electrolysis (DWE)
30.2.1.3 Hybrid Water Electrolysis (HWE)
30.2.1.4 Tandem Water Electrolysis (TWE)
30.2.2 Polymer Electrolyte Membrane Water Electrolysis
30.2.3 Solid Oxide Electrolyte Water Electrolysis
30.3 Electrocatalyst Materials in Liquid Electrolyte Water Splitting. 30.3.1 Oxygen Evolution Reaction Electrocatalysts. 30.3.1.1 Metal Oxides
30.3.1.2 Metal Chalcogenides
30.3.1.3 Metal Pnictides, Carbides, and Borides
30.3.1.4 Metal–Organic Frameworks and Related Materials
30.3.2 Hydrogen Evolution Reaction Electrocatalysts. 30.3.2.1 Non‐noble Metals and Noble Metal–Free Alloys
30.3.2.2 Non‐precious Metal Composites
30.3.2.3 Metal‐Free Electrocatalysts
30.4 Conclusions and Outlook
References
31 New Visible‐Light‐Responsive Photocatalysts for Water Splitting Based on Mixed Anions
31.1 Introduction
31.2 New Doped Rutile TiO2 Photocatalysts for Efficient Water Oxidation
31.3 Unprecedented Narrow‐Gap Oxyfluoride
31.4 Conclusion and Future Perspective
References
32 Electrocatalysts in Polymer Electrolyte Membrane Fuel Cells
32.1 Introduction
32.2 Platinum Electrocatalysts
32.3 Voltammetry
32.4 Cyclic Voltammetry
32.5 Linear Sweep Voltammetry
32.6 Electron Transfer Number
32.7 Durability Measurements in a Three‐Electrode Cell
32.8 Membrane Electrode Assembly (MEA) Fabrication
32.9 MEA Measurements
32.10 Recent Electrocatalyst Research
32.11 Future Perspectives
Acknowledgments
References
33 Conversion of Lignocellulosic Biomass to Biofuels
33.1 Introduction
33.2 Lignocellulosic Biomass: Composition and Resources
33.3 Biofuel Production from Lignocellulosic Biomass
33.3.1 Ethoxymethylfurfural (EMF)
33.3.2 Ethyl Levulinate (EL)
33.3.3 2,5‐Dimethylfuran (DMF)
33.3.4 2‐Methylfuran (MF)
33.3.5 γ‐Valerolactone (GVL)
33.4 Outlook and Conclusions
References
34 Conversion of Carbohydrates to High Value Products
34.1 Introduction
34.2 Overview of Strategy for Catalyst Development and Routes for Conversion of Carbohydrates
34.3 Synthesis of Value‐Added Chemicals from Carbohydrates
34.3.1 Dihydrolevoglucosenone
34.3.2 1,6‐Hexanediol
34.3.3 Furandicarboxylic Acid (FDCA)
34.3.4 Terephthalic Acid
34.3.5 Lactic Acid
34.3.6 Lactide
34.4 Perspective
References
35 Enhancing Sustainability Through Heterogeneous Catalytic Conversions at High Pressure
35.1 Importance of High‐Pressure Reaction Condition
35.1.1 Chemical Equilibrium (One Phase)
35.1.2 Phase Behavior (Multiphase) 35.1.2.1 Phase Separation
35.1.2.2 Supercritical State
35.1.3 Mass Transfer and Kinetics
35.1.3.1 Molecular Diffusion
35.1.3.2 Multiphase Reaction
35.1.4 Process Efficiency and Economy
35.2 State‐of‐the‐Art Application of High Pressure in Heterogeneous Catalysis. 35.2.1 Boosting CO2 Conversion and Surpassing One‐Phase Chemical Equilibrium by In situ Phase Separation at High‐Pressure Reaction Condition
35.2.2 Exploitation of Supercritical Fluid Properties for Catalytic Reactions
35.2.3 A Greener High‐Pressure System Using Microchannel Reactor
35.2.4 Surpassing Chemical Equilibrium by In situ Product Separation Using a High‐Pressure Membrane Reactor
35.3 Concluding Remark
References
36 Electro‐, Photo‐, and Photoelectro‐chemical Reduction of CO2
36.1 Introduction
36.2 Fundamentals. 36.2.1 Redox Processes
36.2.2 Assessment of Reaction Performance: Figures of Merit
36.2.3 Role of Heterogeneous Catalysts
36.3 Innovative Technologies for CO2 Reduction. 36.3.1 Electrochemical Reduction. 36.3.1.1 How Does the Technology Work?
36.3.1.2 Key Factors Influencing Reaction Performance
36.3.1.3 Main Challenges
36.3.2 Photochemical Reduction. 36.3.2.1 How Does the Technology Work?
36.3.2.2 Key Factors
36.3.2.3 Main Challenges
36.3.3 Photoelectrochemical Reduction. 36.3.3.1 How Does the Technology Work?
36.3.3.2 Key Factors
36.3.3.3 Main Challenges
36.4 Concluding Remarks
Acknowledgments
References
37 Photocatalytic Abatement of Emerging Micropollutants in Water and Wastewater
37.1 Introduction
37.2 Main Processes for Photocatalytic Abatement of Micropollutants in Water and Wastewater
37.3 Advancements in Photocatalysts for Photocatalytic Abatement of Micropollutants in Water and Wastewater
37.3.1 Photocatalysts Components Optimization
37.3.1.1 Semiconductor Doping
37.3.1.2 Surface Sensitization
37.3.1.3 Metal Deposition
37.3.1.4 Carbon Materials Combination
37.3.1.5 Combining Semiconductors
37.3.2 Photocatalysts Configuration Optimization. 37.3.2.1 Freestanding Particulate
37.3.2.2 Film, Immobilized, and Aerogel‐Based Catalysts
37.4 Reaction System Optimization. 37.4.1 Reaction Conditions
37.4.2 Solar Reactors
37.5 Future Challenges and Prospects
Acknowledgments
References
38 Catalytic Abatement of NOx Emissions over the Zeolite Catalysts
38.1 Zeolite Catalysts with Different Topologies
38.2 Essential Nature of Novel Cu–CHA catalyst. 38.2.1 Shape Selectivity
38.2.2 Cation Location
38.2.3 Copper Status
38.2.4 CHA‐Type Silicoaluminophosphate
38.3 SCR Reaction Mechanism
38.4 Conclusions and Perspectives
References
Index. a
b
c
d
e
f
g
h
i
j
k
l
m
n
o
p
q
r
s
t
u
v
w
x
y
z
WILEY END USER LICENSE AGREEMENT
Отрывок из книги
Edited by Wey Yang Teoh, Atsushi Urakawa, Yun Hau Ng, and Patrick Sit
Volume 1
.....
In the course of 120 years since the discovery of modern heterogeneous catalysis, almost the entire physical length scale of catalyst design with compositions across the periodic table has been explored. From the use of bulk metals in the form of wires and metal strips during the Faraday era to metal nanoparticles and metal clusters and all the way to SACs, the primary objective has always been to identify the most active, selective, and durable catalysts and at the same time economically feasible (not necessarily low cost) and environmentally benign ones. While the classical techniques for catalyst preparation such as impregnation, precipitation, sol–gel, hydro/solvothermal syntheses, and solid‐state sintering continue to be relevant to this day, both industrially and fundamentally, many new syntheses and design strategies have since emerged. They include electrochemical anodization, supramolecular assembly, microwave synthesis, vapor deposition, spray pyrolysis, flame and plasma synthesis, etc. At the same time, design strategies including those with soft and hard templating to induce highly ordered pore structures, engineering of crystal facets and anisotropy (see Chapter 2 on how they can be creatively used to manipulate the target catalytic reactions), ligand‐capping to obtain well‐defined metal clusters, and surface grafting of single‐atom sites were developed to tune the physicochemical characteristics and hence the reactivities of catalysts. It is such a process of continuously pushing the boundaries of catalyst design that led to many new catalytically usable properties, e.g., size and spatial selective pores, localized surface plasmon resonance, size quantization effects, non‐Newtonian metal–support interactions, and low coordination active sites. The ability to uncover and utilize these new catalytic properties for targeted reactions is what constitutes the frontier in the field.
.....