Substrate-Integrated Millimeter-Wave Antennas for Next-Generation Communication and Radar Systems
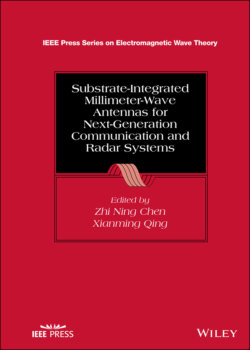
Реклама. ООО «ЛитРес», ИНН: 7719571260.
Оглавление
Группа авторов. Substrate-Integrated Millimeter-Wave Antennas for Next-Generation Communication and Radar Systems
Table of Contents
List of Tables
List of Illustrations
Guide
Pages
IEEE Press
Substrate‐Integrated Millimeter‐Wave Antennas for Next‐Generation Communication and Radar Systems
Editor Biographies
Contributors
Foreword
Preface
1. Introduction to Millimeter Wave Antennas
1.1 Millimeter Waves
1.2 Propagation of Millimeter Waves
1.3 Millimeter Wave Technology. 1.3.1 Important Features
1.3.2 Major Modern Applications
1.3.2.1 Next‐Generation Wireless Communications
1.3.2.2 High‐Definition Video and Virtual Reality Headsets
1.3.2.3 Automotive Communications and Radars
1.3.2.4 Body Scanners and Imaging
1.4 Unique Challenges of Millimeter Wave Antennas
1.5 Briefing of State‐of‐the‐Art Millimeter Wave Antennas
1.6 Implementation Considerations of Millimeter Wave Antennas
1.6.1 Fabrication Processes and Materials of the Antennas
1.6.2 Commonly Used Transmission Line Systems for Antennas
1.7 Note on Losses in Microstrip‐Lines and Substrate Integrated Waveguides
1.8 Update of Millimeter Wave Technology in 5G NR and Beyond
1.9 Organization of the book
1.10 Summary
References
2. Measurement Methods and Setups of Antennas at 60–325 GHz Bands
2.1 Introduction
2.1.1 Far‐Field Antenna Measurement Setup
2.1.1.1 Free‐Space Range Using Anechoic Chamber
2.1.1.2 Compact Range
2.1.2 Near‐Field Antenna Measurement Setup
2.2 State‐of‐the‐Art mmW Measurement Systems. 2.2.1 Commercially Available mmW Measurement Systems
2.2.2 Customized mmW Measurement Systems
2.3 Considerations for Measurement Setup Configuration
2.3.1 Far‐Field versus Near‐Field versus Compact Range. 2.3.1.1 Far‐Field Measurement
2.3.1.2 Near‐Field Measurement
2.3.1.3 Compact Antenna Test Range
2.3.2 RF System
2.3.3 Interface Between the RF Instrument and AUT
2.3.4 On‐Wafer Antenna Measurement
2.3.4.1 Feeding and Movement Limitations
2.3.4.2 Reflection Caused by Probes/Metallic Environment
2.3.4.3 Undesired Coupling Effects Caused by Measurement Probes
2.4 mmW Measurement Setup Examples
2.4.1 60‐GHz Antenna Measurement Setup
2.4.2 140‐GHz Antenna Measurement Setup
2.4.3 270‐GHz Antenna Measurement Setup
2.5 Summary
References
3. Substrate Integrated mmW Antennas on LTCC
3.1 Introduction
3.1.1 Unique Design Challenges and Promising Solutions
3.1.2 SIW Slot Antennas and Arrays on LTCC
3.2 High‐Gain mmW SIW Slot Antenna Arrays on LTCC
3.2.1 SIW Three‐Dimensional Corporate Feed
3.2.2 Substrate Integrated Cavity Antenna Array at 60 GHz
3.2.3 Simplified Designs and High‐Order‐Mode Antenna Array at 140 GHz
3.2.3.1 140‐GHz Slot Antenna Array with a Large‐Via‐Fence Dielectric Loading
3.2.3.2 140‐GHz Slot Antenna Array with a Large‐Via‐Fence and Large‐Slot Dielectric Loading
3.2.3.3 140‐GHz Slot Antenna Array Operating at a Higher‐Order Mode (TE20 Mode)
Design of Pair of Radiating Slots with a Dielectric Loading
Design of E‐Plane Coupler
Design of an 8 × 8 Array
3.2.4 Fully Substrate Integrated Antennas at 270 GHz
3.2.4.1 Analysis of LTCC‐Based Substrate Integrated Structures
Case I: SIW
Case II: Metal Strips at Sidewall
3.2.4.2 Fresnel Zone Plate Antenna in LTCC at 270 GHz
3.3 Summary
References
4. Broadband Metamaterial‐Mushroom Antenna Array at 60 GHz Bands
4.1 Introduction
4.2 Broadband Low‐Profile CRLH‐Mushroom Antenna
4.2.1 Working Principle
4.2.2 Impedance Matching
4.3 Broadband LTCC Metamaterial‐Mushroom Antenna Array at 60 GHz
4.3.1 SIW Fed CRLH‐Mushroom Antenna Element
4.3.2 Self‐Decoupling Functionality
4.3.3 Self‐Decoupled Metamaterial‐Mushroom Subarray
4.3.4 Metamaterial‐Mushroom Antenna Array
4.4 Summary
References
5. Narrow‐Wall‐Fed Substrate Integrated Cavity Antenna at 60 GHz
5.1 Introduction
5.2 Broadband Techniques for Substrate Integrated Antennas
5.2.1 Enhancement of the Impedance Matching for SIW Antennas
5.2.2 Multi‐Mode Substrate Integrated Cavity Antennas
5.2.3 Substrate Integrated Cavity Backed Slot Antenna
5.2.4 Patch Loaded Substrate Integrated Cavity Antenna
5.2.5 Traveling‐Wave Elements Loaded Substrate Integrated Cavity Antenna
5.3 SIW Narrow Wall Fed SIC Antennas at Ka‐ and V‐Bands
5.3.1 SIW Narrow Wall Fed SIC Antenna
5.3.2 SIW Narrow Wall Fed SIC Antenna Array at 35 GHz
5.3.3 60‐GHz SIW Narrow Wall Fed SIC Antenna Array
5.4 Summary
References
6. Cavity‐Backed SIW Slot Antennas at 60 GHz
6.1 Introduction
6.2 Operating Principle of the Cavity‐Backed Antenna. 6.2.1 Configuration
6.2.2 Analysis of the Backing‐Cavity
6.2.3 Design of the Backing‐Cavity
6.3 Cavity‐Backed SIW Slot Antenna
6.3.1 Feeding Techniques
6.3.2 SIW Backing‐Cavity
6.3.3 Radiating Slot
6.4 Types of SIW CBSAs. 6.4.1 Wideband CBSAs
6.4.2 Dual‐Band CBSAs
6.4.3 Dual‐Polarized and Circularly Polarized CBSAs
6.4.4 Miniaturized CBSAs
6.5 CBSA Design Examples at 60 GHz
6.5.1 SIW CBSA with Different Slot WLR
6.5.2 Array Examples with Different WLRs of Slot
6.6 Summary
References
7. Circularly Polarized SIW Slot LTCC Antennas at 60 GHz
7.1 Introduction
7.2 Key Techniques of mmW CP Antenna Array
7.2.1 Antenna Element Selection
7.2.2 AR Bandwidth Enhancement Methods
7.3 Wideband CP LTCC SIW Antenna Array at 60 GHz
7.3.1 Wideband AR Element
7.3.2 Isolation Consideration
7.3.3 Experiment Results and Discussion
7.4 Summary
References
8. Gain Enhancement of LTCC Microstrip Patch Antenna by Suppressing Surface Waves
8.1 Introduction. 8.1.1 Surface Waves in Microstrip Patch Antennas
8.1.2 Surface Waves Effects on Microstrip Patch Antenna
8.2 State‐of‐the‐Art Methods for Suppressing Surface Waves in Microstrip Patch Antennas
8.3 Microstrip Patch Antennas with Partial Substrate Removal
8.3.1 Technique of Partial Substrate Removal
8.3.2 60‐GHz LTCC Antenna with Partial Substrate Removal
8.4 Summary
References
9. Substrate Integrated Antennas for Millimeter Wave Automotive Radars
9.1 Introduction
9.1.1 Automotive Radar Classification
9.1.2 Frequency Bands for Automotive Radars
9.1.3 Comparison of 24 GHz and 77 GHz Bands
9.1.4 Antenna System Considerations for Automotive Radar Sensors
9.1.4.1 Lens Antenna and Reflector Antenna
9.1.4.2 Planar Antennas
9.1.5 Fabrication and Packaging Considerations
9.2 State‐of‐the‐Art Antennas for 24‐GHz and 77‐GHz Automotive Radars
9.2.1 Selected State‐of‐the‐Art Antennas for 24‐GHz Automotive Radars. 9.2.1.1 Shorted Parasitic Rhombic Patch Antenna Array with Lower Cross‐Polarization Levels
9.2.1.2 Compact Two‐Layer Rotman Lens‐Fed Microstrip Antenna Array
9.2.1.3 SIW Parasitic Antenna Array Without Feeding Network
9.2.1.4 SIW Pillbox Antenna Integrating Monopulse Amplitude‐Comparison Technique
9.2.2 Selected State‐of‐the‐Art Antennas for 77‐GHz Automotive Radars. 9.2.2.1 SIW Slot Array for Both Medium‐ and Long‐Range Automotive Radar Sensor
9.2.2.2 16 × 16 Phased Array Antenna/Receiver Packaged Using Bond‐Wire Technique
9.2.2.3 Antenna/Module in Package
9.3 Single‐Layer SIW Slot Antenna Array for 24‐GHz Automotive Radars
9.3.1 Antenna Configuration
9.3.2 Slot Array Design
9.3.3 Feeding Network Design
9.3.4 Experiment Results
9.4 Transmit‐Array Antenna for 77‐GHz Automotive Radars
9.4.1 Unit Cell
9.4.2 Four‐Beam Transmit‐Array
9.4.3 Results
9.5 Summary
Acknowledgments
References
10. Sidelobe Reduction of Substrate Integrated Antenna Arrays at Ka‐Band
10.1 Introduction
10.2 Feeding Networks for Substrate Integrated Antenna Array
10.2.1 Series Feeding Network
10.2.2 Parallel/Corporate Feeding Network
10.2.3 Flat Lens/Reflector‐Based Quasi‐Optics Feeding Network
10.2.4 Power Dividers
10.3 SIW Antenna Arrays with Sidelobe Reduction at Ka‐Band
10.3.1 Double‐Layer 8 × 8 SIW Slot Array
10.3.1.1 Parameter Extraction of Radiating Slots
10.3.1.2 Feeding Network
10.3.1.3 Simulations and Experiments
10.3.2 16 × 16 Monopulse SIW Slot Array
10.3.2.1 Series T‐Junction Feeding Network
10.3.2.2 Sum‐Difference Network
10.3.2.3 Simulations and Experiments
10.4 Summary
References
11. Substrate Edge Antennas
11.1 Introduction
11.2 State‐of‐the‐Art. 11.2.1 End‐Fire SEAs
11.2.2 Leaky‐Wave SEAs
11.3 Tapered Strips for Wideband Impedance Matching
11.3.1 Tapered Triangular Strips
11.3.2 Tapered Rectangular Strips
11.4 Embedded Planar Lens for Gain Enhancement
11.4.1 Embedded Metallic Lens
11.4.2 Embedded Gap Lens
11.5 Prism Lens for Broadband Fixed‐Beam Leaky‐Wave SEAs
11.6 Summary
References
Index. a
b
c
d
e
f
g
h
i
k
l
m
n
o
p
q
r
s
t
u
v
w
y
IEEE Press Series on Electromagnetic Wave Theory
WILEY END USER LICENSE AGREEMENT
Отрывок из книги
445 Hoes Lane
Piscataway, NJ 08854
.....
With an LTCC process, the LTCC ceramic substrate can host almost an infinite number of layers. The thin layers are stacked one on the top of another. The conducting paths of gold or silver thick film pastes are printed on each surface layer by layer using the silk‐screen printing method. When the multilayer setup has been stacked and printed, it is then fired in the process oven where the low sintering temperature allows the use of gold and silver as conducting traces. The simplified description of process includes:
The PCB and LTCC processes are concisely compared in Figure 1.5. From a waveguide feeding network and antenna design point of view, the most important difference between the PCB and LTCC processes is that the LTCC process is able to implement the blind via and embedded cavity while the PCB process is unable to do it.
.....