Mantle Convection and Surface Expressions
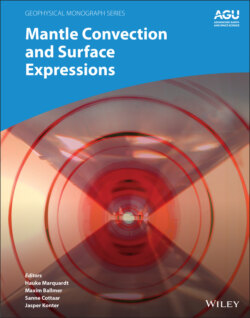
Реклама. ООО «ЛитРес», ИНН: 7719571260.
Оглавление
Группа авторов. Mantle Convection and Surface Expressions
Table of Contents
List of Tables
List of Illustrations
Guide
Pages
Geophysical Monograph Series
Geophysical Monograph 263. Mantle Convection and Surface Expressions
Dedication
LIST OF CONTRIBUTORS
PREFACE
Part I: State of the Mantle: Properties and Dynamic Evolution
Part II: Material Transport Across the Mantle: Geophysical Observations and Geodynamic Predictions
Part III: Surface Expressions: Mantle Controls on Planetary Evolution and Habitability
1 Long‐Wavelength Mantle Structure: Geophysical Constraints and Dynamical Models
ABSTRACT
1.1 INTRODUCTION
1.2 METHODS. 1.2.1 Mantle Tomography
1.2.2 Mantle Circulation Models
1.2.3 Inversions for Viscosity
1.3 RESULTS
1.4 DISCUSSION
1.5 CONCLUSIONS
ACKNOWLEDGMENTS
REFERENCES
2 Experimental Deformation of Lower Mantle Rocks and Minerals
ABSTRACT
2.1 INTRODUCTION
2.2 BACKGROUND
2.3 METHODS: EXPERIMENTAL DEFORMATION OF LOWER MANTLE MINERALS
2.3.1 The Diamond Anvil Cell as a Deformation Device
2.3.2 Large‐Volume Deformation Devices
2.3.3 Texture and Strength Measurements in High‐Pressure Experiments
2.3.4 A Note on Scaling Experiments to the Lower Mantle
2.4 DEFORMATION STUDIES OF LOWER MANTLE PHASES
2.4.1 Differential Stress Measurements in Lower Mantle Phases
Ferropericlase
CaSiO3 Perovskite
Bridgmanite
Post‐Perovskite
2.4.2 Textures and Slip Systems in Lower Mantle Phases
Ferropericlase
CaSiO3 Perovskite
Deformation of Bridgmanite
2.4.3 Post‐Perovskite
2.5 POLYPHASE DEFORMATION
2.5.1 Stress and Strain Partitioning in Polyphase Aggregates
2.5.2 Differential Stresses in High Pressure Studies of Polyphase Aggregates
2.5.3 Texture Development in Polyphase Materials
2.5.4 Texture Development in High Pressure Studies of Polyphase Aggregates
2.6 IMPLICATIONS
2.7 CONCLUSIONS
ACKNOWLEDGMENTS
REFERENCES
3 Seismic Wave Velocities in Earth’s Mantle from Mineral Elasticity
ABSTRACT
3.1 INTRODUCTION
3.2 ELASTIC PROPERTIES
3.3 EXPERIMENTS
3.4 COMPUTATIONS
3.5 PARAMETER UNCERTAINTIES
3.6 ELASTIC PROPERTIES OF SOLID SOLUTIONS
3.7 ELASTIC ANOMALIES FROM CONTINUOUS PHASE TRANSITIONS
3.8 EARTH’S LOWER MANTLE
3.9 CONCLUSIONS
ACKNOWLEDGMENTS
REFERENCES
4 From Mantle Convection to Seismic Observations: Quantifying the Uncertainties Related to Anelasticity
ABSTRACT
4.1 INTRODUCTION
4.2 FROM GEODYNAMIC HYPOTHESIS TO SEISMIC OBSERVATIONS
4.2.1 Predicting Seismic Mantle Structure
Mantle Evolution and the Present‐Day Temperature Field
Relating Temperatures to Seismic Velocities
4.2.2 Predicting Seismic Observables – Truly “Synthetic” Data
4.2.3 Linking to Earth Observations – The Importance of Uncertainties
4.3 CONSIDERING MINERALOGICAL UNCERTAINTIES
4.3.1 Anelasticity – A Crucial Factor for Traveltime Uncertainty
Frequency and Temperature Dependence of Q
Anelasticity Correction of Seismic Velocities
4.4 QUANTIFYING THE EFFECTS OF UNCERTAINTIES RELATED TO ANELASTICITY
4.5 SUMMARY AND CONCLUDING REMARKS
ACKNOWLEDGMENTS
REFERENCES
5 Geochemical Diversity in the Mantle
ABSTRACT
5.1 INTRODUCTION
5.2 DEFINITIONS AND INTERPRETATIONS OF DISTINCT MANTLE COMPONENTS
5.2.1 Depleted Mantle Component
5.2.2 Enriched Mantle Component
5.2.3 HIMU Mantle Component
5.2.4 FOZO and Primordial Mantle Components
5.3 LITHOLOGICAL ASPECTS OF THE MANTLE COMPONENTS
5.4 THE ROLE OF CARBONATE IN MANTLE GEOCHEMICAL HETEROGENEITY
5.5 RECENT PROGRESS AND OUTLOOK FOR THE MANTLE EVOLUTION
5.5.1 Nontraditional Stable Isotopes as New Proxies to Trace Recycling
5.5.2 Volatile Recycling
5.5.3 Ancient Surface Environments and Mantle Evolution
ACKNOWLEDGMENTS
REFERENCES
6 Tracking the Evolution of Magmas from Heterogeneous Mantle Sources to Eruption
ABSTRACT
6.1 INTRODUCTION
6.2 PARTIAL MELTING OF A HETEROGENEOUS MANTLE. 6.2.1 Contribution of Mantle Lithologies to Magma Genesis
6.3 PERIDOTITE VS. PYROXENITE: EXPERIMENTAL MELT COMPOSITIONS. 6.3.1 Major Elements
6.3.2 First Row Transition Elements
6.4 INTERACTIONS BETWEEN CHEMICAL HETEROGENEITIES AND PERIDOTITE
6.5 SAMPLING MELTS OF THE MANTLE: APPROACH AND OVERVIEW
6.5.1 MORBs. Intracrustal Processing
Natural MORBs and Their Cumulates
Primary MORBs
6.5.2 Ocean Island Basalts
Source Lithologies of Primitive OIBs
Natural OIB and Their Cumulates
6.5.3 Arc Magmas. Arc Magma Genesis – A Complexity of Factors
Experimental Perspectives on Primary Arc Magma Generation: Melting of the Peridotitic Mantle Wedge
Melting of Nonperidotitic Sources in Arc Magma Generation. Downgoing plate contributions to fluid flux and sediment melt diapirs
Upper plate processes
Natural Arc Magmas and Their Cumulates
6.6 FUTURE DIRECTIONS
ACKNOWLEDGMENTS
REFERENCES
7 Super‐Deep Diamonds: Emerging Deep Mantle Insights from the Past Decade
ABSTRACT
7.1 INTRODUCTION
7.2 OCEAN CRUST AND CARBON RECYCLED TO THE LOWER MANTLE
7.3 RECOGNIZING CARBONATITIC MELT SIGNATURES IN THE DEEP MANTLE
7.4 SIGNS OF DEEP WATER
7.5 PRESERVED DEEP MANTLE MINERALS WITH ORIGINAL CRYSTAL STRUCTURE
7.6 NEW KINDS OF SUPER‐DEEP DIAMONDS: CLIPPIR AND TYPE IIB DIAMONDS
7.7 A REDUCED IRON‐SATURATED SUBLITHOSPHERIC MANTLE
7.8 DISCUSSION
ACKNOWLEDGMENTS
REFERENCES
8 Seismic and Mineral Physics Constraints on the D" Layer
ABSTRACT
8.1 INTRODUCTION
8.2 THE D" REFLECTOR(S) 8.2.1 Visibility of the Reflection
8.2.2 Travel Times of the Reflection(s)
8.2.3 Amplitude and Polarity of the Reflection(s)
8.2.4 Plausible Explanations
8.2.5 Case Study: Observations and Interpretations of D" Underneath the Northern Pacific Region
8.3 ULTRALOW VELOCITY ZONES. 8.3.1 Seismic Observations
8.3.2 Plausible Explanations
Partial Melt
Extreme Chemical or Phase Heterogeneity
Anisotropy
8.3.3 Inversion of Iron‐Rich Phase Assemblages with Seismic Observations
ULVZ Case 1: Europe and Western Asia
ULVZ Case 2: NW America
ULVZ Case 3: Central America
ULVZ Case 4: Coral Sea
ULVZ Case 5: Anisotropy
8.3.4 ULVZ Topography
8.3.5 Summary of ULVZ Case Studies
8.4 SUMMARY AND CONCLUSIONS
ACKNOWLEDGMENTS
APPENDIX
REFERENCES
9 Toward Consistent Seismological Models of the Core–Mantle Boundary Landscape
ABSTRACT
9.1 INTRODUCTION
9.2 EXISTING MODELS OF CMB TOPOGRAPHY
9.2.1 Regional CMB Topography Studies
9.2.2 Global Body‐Wave Models of CMB Topography
9.2.3 CMB Topography Constrained by Normal Modes
9.2.4 Other Constraints on CMB Topography
9.2.5 Dynamic Predictions of CMB Topography
9.3 TWENTY YEARS OF DENSITY MODELS
9.3.1 Early Normal‐Mode Studies
9.3.2 Recent Studies Utilizing Improved Data Sets
9.3.3 New Developments in Density Studies
9.4 QUANTITATIVE ASSESSMENT OF EXISTING SEISMOLOGICAL MODELS
9.4.1 Cross‐Model Correlation
9.4.2 Average Models
9.4.3 Vote Maps of Density and CMB Topography
9.4.4 Comparison to Geodynamic Predictions
9.4.5 Summary of Current Model Features
9.5 EFFORTS TOWARD MORE CONSISTENT MODELS
9.5.1 Factors Influencing the Interpretation of Density Models
9.5.2 Future Development of Density Models
9.5.3 Developing Consistent CMB Topography Models
9.5.4 Integrating Seismology With Other Constraints
9.6 CONCLUSIONS
ACKNOWLEDGMENTS
REFERENCES
10 Dynamics of the Upper Mantle in Light of Seismic Anisotropy
ABSTRACT
10.1 INTRODUCTION
10.2 OBSERVATIONS OF SEISMIC ANISOTROPY
10.2.1 Pn Anisotropy
10.2.2 Shear Wave Splitting
10.2.3 Surface Waves
10.3 INTERPRETATION OF SEISMIC ANISOTROPY. 10.3.1 Origin of Upper Mantle Anisotropy
10.3.2 Anisotropy and Plate Motions
10.3.3 Mantle Circulation Modeling
Boundary Layer Anisotropy
10.3.4 Examples of Inferences That Extend Beyond the Reference Model
10.4 OPEN QUESTIONS. 10.4.1 Regional Complexities and Scale‐Dependent Resolution
Oceanic Plates Revisited
Anisotropy in Continents
10.4.2 Uncertainties About Microphysics. Formation of Olivine LPO
Mechanical Anisotropy
10.5 WAYS FORWARD
10.6 CONCLUSIONS
ACKNOWLEDGMENTS
REFERENCES
11 Mantle Convection in Subduction Zones: Insights from Seismic Anisotropy Tomography
ABSTRACT
11.1 INTRODUCTION
11.2 METHOD
11.2.1 Azimuthal Anisotropy Tomography
11.2.2 Radial Anisotropy Tomography
11.3 APPLICATIONS
11.3.1 Northwest Pacific
11.3.2 Southeast Asia
11.3.3 New Zealand
11.3.4 Alaska
11.3.5 North America
11.3.6 Europe
11.4 DISCUSSION. 11.4.1 Surface‐Wave Anisotropic Tomography
11.4.2 Shear‐Wave Splitting Tomography
11.4.3 Anisotropic Tomography: What Can We Learn and Expect?
11.5 CONCLUSIONS
ACKNOWLEDGMENTS
REFERENCES
12 The Cycling of Subducted Oceanic Crust in the Earth’s Deep Mantle
ABSTRACT
12.1 INTRODUCTION
12.2 OBSERVATIONAL CONSTRAINTS ON THE DISTRIBUTION OF OCEANIC CRUST IN THE DEEP MANTLE. 12.2.1 Geochemical Observations
12.2.2 Seismic Observations
12.3 THE CYCLING OF SUBDUCTED OCEANIC CRUST IN THE DEEP MANTLE
12.3.1 The Separation of Slab Components at the Base of Transition Zone
12.3.2 The Accumulation of Subducted Oceanic Crust on the CMB
12.3.3 Stability and Entrainment of the Crustal Accumulations on the CMB
12.3.4 The Fate of the Entrained Oceanic Crust
12.4 SUMMARY AND FUTURE WORK
12.5 ACKNOWLEDGMENTS
REFERENCES
13 Toward Imaging Flow at the Base of the Mantle with Seismic, Mineral Physics, and Geodynamic Constraints
ABSTRACT
13.1 INTRODUCTION
13.2 OBSERVATIONAL CONSTRAINTS ON LOWERMOST MANTLE FLOW. 13.2.1 Global Tomographic Models
13.2.2 Regional Body‐Wave Observations
13.2.3 Observed Regional Anisotropy
13.3 FORWARD MODELING
13.3.1 Geodynamic Models
13.3.2 Mineralogical Constraints
Bridgmanite
Post‐perovskite
Ferropericlase
Other Phases
13.4 JOINT GEODYNAMIC–SEISMIC MODELING
13.4.1 Recent Developments
13.4.2 Example Case: Comparing a Model to Seismic Observations
Geodynamic and Texture Modeling
Seismic Modeling
Synthetic Analysis
13.5 LIMITATIONS, ADVANCES, AND THE WAY FORWARD. 13.5.1 The Inverse Problem
13.5.2 Outlook
13.6 ACKNOWLEDGMENTS
REFERENCES
14 Seismic Imaging of Deep Mantle Plumes
ABSTRACT
14.1 INTRODUCTION
14.2 PHYSICAL MODELS OF DEEP MANTLE PLUMES
14.3 TRAVELTIME ANALYSIS
14.3.1 Expected Traveltime Delays
14.3.2 Synthetic Tomography of Deep Mantle Plumes
14.4 UNDULATIONS OF THE 410‐KM AND 660‐KM PHASE TRANSITIONS
14.5 DIFFRACTION AND SCATTERING EFFECTS
14.6 CONCLUDING REMARKS
ACKNOWLEDGMENTS
REFERENCES
15 Observational Estimates of Dynamic Topography Through Space and Time
ABSTRACT
15.1 INTRODUCTION
15.2 CONVECTIVE REGIMES AND THEIR SURFACE EXPRESSION
15.2.1 Thermal Convection of the Mantle
15.2.2 Dynamic Topography: A Definition
15.2.3 Simple Convection Simulations
15.3 EARLY OBSERVATIONAL CONSTRAINTS ON LARGE‐SCALE MANTLE FLOW AND THEIR ROLE IN PAST AND PRESENT GEODYNAMIC MODELING
15.4 ESTIMATING PRESENT‐DAY DYNAMIC TOPOGRAPHY
15.4.1 Oceanic Residual Depth Anomalies
15.4.2 Continental Residual Topography
15.5 OBSERVATIONAL ESTIMATES OF TIME‐EVOLVING DYNAMIC TOPOGRAPHY
15.5.1 Satellite Observations
15.5.2 Sea‐Level Markers
15.5.3 Sedimentary Flux and Stratal Architecture of Basins
15.5.4 Peneplanation and Palaeosurfaces
15.5.5 Landscape Denudation and Thermochronology
15.5.6 Drainage Analysis
15.5.7 Paleoaltimetry
15.5.8 Bathymetric Indicators
15.6 FRONTIERS AND OUTSTANDING CHALLENGES
15.6.1 Continental Residual Topography
15.6.2 Integrated Landscape Evolution Analysis
15.6.3 Spectral Properties of Dynamic Topography
15.6.4 Flow Regime in the Asthenosphere
15.6.5 Numerical Convection Models and Rheology of the Upper Mantle
15.7 SUMMARY
ACKNOWLEDGMENTS
REFERENCES
16 Connecting the Deep Earth and the Atmosphere
ABSTRACT
16.1 INTRODUCTION
16.2 OBSERVED LINKS BETWEEN DEEP EARTH AND SURFACE VOLCANISM
16.3 PLUME ASCENT SCENARIOS
16.4 VOLCANISM AND ENVIRONMENTAL EFFECTS ON VARIOUS TIMESCALES
16.4.1 Kimberlites
16.4.2 Large Igneous Provinces and Global Changes
16.5 LIPs AND PALEOGEOGRAPHY: LONG‐TERM EFFECTS
16.5.1 Plate Tectonic Configurations and Their Modifications by LIPs
16.5.2 Paleoclimate and Atmospheric CO2
16.5.3 Paleolatitude of LIP Emplacement
16.5.4 GEOCARBSULF: Proxy‐Model CO2 Mismatches
16.6 CONCLUDING REMARKS AND CHALLENGES
ACKNOWLEDGMENTS
REFERENCES
APPENDIX 16.1
17 Mercury, Moon, Mars: Surface Expressions of Mantle Convection and Interior Evolution of Stagnant‐Lid Bodies
ABSTRACT
17.1 INTRODUCTION
17.2 MAGMA OCEAN SOLIDIFICATION AND ONSET OF SOLID‐STATE MANTLE CONVECTION
17.2.1 Late Onset of Mantle Convection and Global Overturn
17.2.2 Early Onset of Mantle Convection and Progressive Mixing
17.3 CRUSTAL MANIFESTATIONS
17.3.1 Primary Crusts
17.3.2 Volume and Time of Emplacement of the Secondary Crust
17.4 IMPACTS
17.4.1 Basin Formation
17.4.2 Impact‐Induced Melting
17.4.3 Impact‐Related Effects on Global Mantle Dynamics
17.4.4 Local Signatures of Impact‐Related Effects
17.5 MANTLE COOLING AND MAGNETIC FIELD GENERATION
17.5.1 Mercury’s Present‐Day and Early Magnetic Field
17.5.2 Mars’s Early Magnetic Field
17.5.3 The Long‐Lived Lunar Magnetic Field
17.6 ADDITIONAL SURFICIAL MANIFESTATION
17.6.1 Global Contraction and Expansion
17.6.2 Heat Flux and Elastic Lithosphere Thickness
17.7 SUMMARY AND OUTLOOK
ACKNOWLEDGMENTS
REFERENCES
NOTE
INDEX
WILEY END USER LICENSE AGREEMENT
Отрывок из книги
Hauke Marquardt Maxim Ballmer Sanne Cottaar Jasper Konter
.....
Figure 2.5 Summary of the various textures types observed in post‐perovskite structured compounds over a range of pressure and temperature conditions. Textures are represented as equal area, upper hemisphere projection, inverse pole figures of the compression direction. Scale bar is in multiples of random distribution.
Evidence for (100) or and/ or {110}〈10〉 comes from theoretical calculations and two early DAC studies. First‐principles metadynamics and energetics of stacking faults predicted slip on {110}〈10〉 during the phase transformation from Brg to pPv (Oganov et al., 2005). Shortly thereafter, room temperature radial diffraction DAC experiments on MgGeO3 pPv and MgSiO3 pPv found textures characterized by (100) planes at high angles to compression (Figure 2.5b, c). Polycrystal plasticity modeling showed that these texture could be explained by slip on {110}〈10〉 and (100) planes (Merkel et al., 2006, 2007). It is important to note that both Merkel et al. (2006) and Merkel et al. (2007) converted to the pPv phase directly from the enstatite phase, and no change in texture was observed upon further pressure increase. Subsequent studies on MgGeO3 pPv in the DAC using axial diffraction geometry (Okada et al., 2010) and radial diffraction geometry (Miyagi et al., 2011) found that alignment of (100) planes at high angles to compression resulted from the phase transformation from an enstatite starting material (Figure 2.5d). Further compression showed that the initial (100) transformation texture shifted to (001) (Figure 2.5e) upon deformation consistent with dominant slip on (001)[100] (Table 1; Miyagi et al., 2011; Okada et al., 2010).
.....