Spectroscopy for Materials Characterization
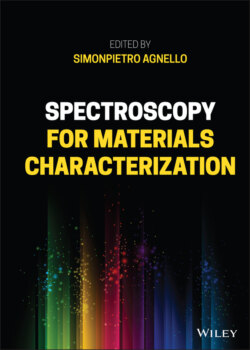
Реклама. ООО «ЛитРес», ИНН: 7719571260.
Оглавление
Группа авторов. Spectroscopy for Materials Characterization
Table of Contents
List of Tables
List of Illustrations
Guide
Pages
Spectroscopy for Materials Characterization
Preface
List of Contributors
1 Radiation–Matter Interaction Principles: Optical Absorption and Emission in the Visible‐Ultraviolet Region
1.1 Empirical Aspects of Radiation–Matter Interaction
1.1.1 Optical Absorption: The Lambert–Beer Law
1.1.2 Emission: Fluorescence and Phosphorescence
1.2 Microscopic Point of View
1.2.1 Einstein Coefficients
1.2.2 Oscillator Strength, Lifetime, Quantum Yield
1.2.3 Vibronic States: Homogeneous and Inhomogeneous Lineshape
1.2.4 Jablonski Energy Level Diagram: Permitted and Forbidden Transitions
1.2.5 Excited States Rate Equations
1.3 Instrumental Setups
1.3.1 Typical Block Diagram of Spectrometers
1.3.2 Light Sources
1.3.3 Dispersion Elements: Gratings and Resolution Power
1.3.4 Detectors: Photodiode, Photomultiplier, Charge Coupled Device
1.4 Case Studies
1.4.1 Optical Absorption in Visible‐Ultraviolet Range
1.4.1.1 Scanning Device (Bandwidth and Scanning Speed Effects)
1.4.1.2 CCD Fiber Optic Device
1.4.2 Photoluminescence
1.4.2.1 Emission and Excitation Spectra: Energy Levels Reconstruction
References
2 Time‐Resolved Photoluminescence
2.1 Introduction to Photoluminescence Spectroscopy
2.1.1 Photoluminescence Properties Related to Points Defects: Electron–Phonon Coupling
2.1.2 Optical Transitions: The Franck–Condon Principle
2.1.3 Zero‐Phonon Line
2.1.4 Phonon Line Structure
2.1.5 Vibrational Structure
2.1.6 Inhomogeneous Effects
2.2 Experimental Methods and Analysis. 2.2.1 Time‐Resolved Luminescence
2.2.2 Site‐Selective Luminescence
2.2.3 Basic Design of Experimental Setup: Pulsed Laser Sources; Monochromators; Detectors
2.2.3.1 Tunable Laser
2.2.3.2 Time‐Resolved Detection System: Spectrograph and Intensified CCD Camera
2.3 Case Studies: Luminescent Point Defects in Amorphous SiO2
2.3.1 Emission Spectra and Lifetime Measurements
2.3.2 Zero‐Phonon Line Probed by Site‐Selective Luminescence
References
3 Ultrafast Optical Spectroscopies
3.1 Femtosecond Spectroscopy: An Overview
3.2 Ultrafast Optical Pulses. 3.2.1 General Properties
3.2.1.1 Dispersion Effect: Group Velocity Dispersion
3.2.2 Nonlinear Optics: Basis and Applications. 3.2.2.1 Second Harmonic Generation and Sum Frequency Generation
3.2.2.2 Noncollinear Optical Parametric Amplifier
3.2.2.3 Supercontinuum Generation
3.3 Transient Absorption Spectroscopy
3.3.1 The Experimental Method
3.3.2 Typical Experimental Setups
3.3.3 Data Analysis and Interpretation
3.4 Ultrafast Fluorescence Spectroscopies
3.4.1 FLUC: The Experimental Method
3.4.2 FLUC: Typical Experimental Setups
3.4.3 FLUC: Data Analysis and Interpretation
3.4.4 Kerr‐Based Femtosecond Fluorescence Spectroscopy
3.5 Femtosecond Stimulated Raman Spectroscopy
3.5.1 The Experimental Method
3.5.2 Typical Experimental Setups
3.5.3 Data Analysis and Interpretation
3.6 Case Studies. 3.6.1 Ultrafast Relaxation Dynamics of Molecules in Solution Phase
3.6.2 Relaxation of Excited Charge Carriers and Excitons in Semiconductor Nanoparticles
3.6.3 Ultrafast Relaxation Dynamics of Carbon‐based Nanomaterials
References
4 Confocal and Two‐Photon Spectroscopy
4.1 Introduction and Historical Perspectives
4.1.1 Point Spread Function and Optical Resolution
4.1.2 Optical Sectioning and Imaging of 3D Samples
4.2 Fluorescence Imaging
4.2.1 Laser Scanning Confocal Fluorescence Microscope
4.2.2 Two‐Photon Microscope
4.2.3 The Importance of Sample Preparation from Solid State to Dynamic Specimens
4.2.4 Setting Up a Measurement
4.3 Spectroscopy Using a Microscope
4.3.1 Observables in Fluorescence Microscopy
4.3.2 Measuring Dynamics: Gaining Information Below Resolution
4.4 Case Studies
4.4.1 Understanding Microstructures and Mechanistic Aspects in Materials
4.4.2 Fluctuation Methods for the Analysis of Nanosystems
References
5 Infrared Absorption Spectroscopy
5.1 Fundamentals
5.1.1 Introduction
5.1.2 Basic Principles
5.1.3 Infrared Spectra
5.1.4 Fourier Transform Infrared Spectrometers (Interferometers)
5.2 Sources and Detectors
5.3 Techniques and Sampling Methods
5.3.1 Transmission Methods
5.3.1.1 Solid Samples
5.3.1.2 Liquid and Solution Samples
5.3.1.3 Gas Samples
5.3.2 Attenuated Total Reflectance (ATR) Method
5.3.3 FTIR Microspectroscopy
5.3.4 AFM‐IR Spectroscopy
5.3.5 Hyphenated Techniques
5.4 Applications and Case Studies
5.4.1 Chemical Characterization and Kinetics
5.4.2 Surfaces
5.4.3 Medical and Life Science (Pharmaceutical, Medical, Biological, Biotechnological)
5.4.4 Cultural Heritage and Forensic
5.4.5 Environmental and Geological
5.4.6 Food Industry
References
6 Raman and Micro‐Raman Spectroscopy
6.1 Basic Theory
6.1.1 Introduction
6.1.2 Spectroscopic Units
6.1.3 Molecular Vibrations
6.1.4 Classical Theory of the Raman Scattering
6.1.5 Simplified Quantum Approach to Raman Scattering
6.1.6 Raman and IR Activities
6.1.7 Crystal Vibrations
6.1.8 Raman Scattering in Crystals
6.1.9 Surface‐Enhanced Raman Scattering (SERS)
6.2 Instrumentation
6.2.1 Laser Sources and Optical Filters
6.2.2 Monochromators
6.2.3 Detectors
6.2.4 Raman Microscopy and Raman Mapping
6.3 Case Studies
6.3.1 Raman Indicators
6.3.2 Identification of Materials and Crystalline Quality
6.3.3 Graphene and Graphite Raman Spectra
6.3.4 Doping Detection
6.3.5 Basic Examples of SERS
References
Note
7 Thermally Stimulated Luminescence
7.1 Theory of Thermally Stimulated Luminescence
7.1.1 Simple Model
7.1.1.1 First‐Order Kinetics
7.1.1.2 Second‐Order Kinetics
7.1.1.3 General‐Order Kinetics
7.1.2 Localized Transitions
7.1.3 Beyond the Ideal Behavior
7.1.3.1 Luminescence Quenching
7.1.3.2 Trap Energy Distributions
7.2 Data Analysis Methods
7.2.1 Initial Rise
7.2.2 Peak Shape
7.2.3 Heating Rate Method
7.2.4 Glow Curve Fit
7.3 Instrumentation and Considerations on Samples
7.4 Case Studies
7.4.1 Lanthanide Energy Level Position in the Bandgap
7.4.2 Bandgap Engineering
7.4.3 Correlation of TSL Data with EPR Results
Note
References
8 Spectroscopic Studies of Radiation Effects on Optical Materials
8.1 Introduction
8.1.1 Radiation Environments
8.1.2 Applications for Optical Materials
8.2 Radiation‐Induced Effects on Optical Materials and Optical Fibers
8.2.1 Radiation‐Induced Attenuation – RIA
8.2.2 Radiation‐Induced Emission – RIE
8.2.3 Radiation‐Induced Compaction – RIC and Refractive Index Change – RIRIC
8.2.4 Origins of Radiation‐Induced Optical Changes
8.3 Radiation‐Induced Attenuation Measurements
8.3.1 Postirradiation RIA Measurements
8.3.1.1 Bulk Glasses
8.3.1.2 Optical Fibers
8.3.2 In Situ RIA Measurements
8.3.2.1 Bulk Glasses
8.3.2.2 Optical Fibers
8.3.2.2.1 Steady State Irradiation
8.3.2.2.2 Pulsed Irradiation
8.3.2.2.3 Hints for Particular Environments, Applications
X‐Ray Testing/Principle
X‐Ray Testing/Implementation
X‐Ray Testing/Advantages and Limitations
γ‐Ray Testing/Principle
γ‐Ray Testing/Implementation
γ‐Ray Testing/Advantages and Limitations
Other Radiation Facilities
8.3.3 Exploitation of RIA Spectra: Point Defect Identification
8.3.3.1 Spectral Decomposition
8.3.3.2 Point Defect Kinetics
8.4 Radiation‐Induced Luminescence (RIL)
8.4.1 Architectures of Fiber‐Based Sensors: Extrinsic and Intrinsic
8.4.2 Calibration of the RIL Versus Proton Flux
8.4.3 Bragg Peak Measurements for Proton‐Therapy Applications
8.5 Case Studies
8.5.1 Characterization of Bulk Glasses for Space Optical Systems
8.5.2 Fiber‐Based Dosimetry with Phosphorus‐Doped Optical Fibers
8.5.3 Proton Flux Measurements Through the RIL of Optical Fibers
References
9 Electron Paramagnetic Resonance Spectroscopy (EPR)
9.1 Introduction
9.2 Basic Principle of EPR
9.3 Anisotropy of g and Spectral Lineshape
9.4 The EPR Lineshape in Powder or in Amorphous
9.5 Hyperfine Interactions
9.6 Paramagnetic Center with S = 1
9.7 Basics of Continuous Wave EPR Setup
9.8 Parameters for EPR Signal Acquisition
9.9 Cw EPR Case Studies
9.10 Time‐Resolved EPR Spectroscopy
9.10.1 Saturation Transients
9.10.2 Spin Nutations
9.10.3 Free Induction Decay
9.10.4 Spin Echo
References
10 Nuclear Magnetic Resonance Spectroscopy
10.1 Introduction
10.2 NMR General Concepts. 10.2.1 Nuclear Spin and Magnetic Moment
10.2.2 Spin Precession and Larmor Frequency
10.2.3 Longitudinal Magnetization
10.2.4 Transverse Magnetization and NMR Signal
10.2.5 Spin Interactions
10.2.6 Fourier Transform NMR
10.3 Liquid‐State NMR. 10.3.1 The NMR Spectrometer
10.3.2 Sample Preparation
10.3.3 How to Set an Experiment
10.3.4 Longitudinal Relaxation Time Measurement
10.3.5 Transverse Relaxation Time Measurement
10.3.6 2D‐Liquid‐State NMR Techniques
10.3.7 Considerations on the Molecular Dynamics by NMR Spectroscopy
10.4 Solid‐State NMR. 10.4.1 Powdered Samples
10.4.2 Cross‐Polarization and Heteronuclear Decoupling
10.4.3 Magic‐Angle Spinning
10.4.4 Homonuclear Dipolar Decoupling
10.4.5 2D‐Solid State NMR Techniques
10.4.6 Recoupling Techniques
10.4.7 Molecular Dynamics by Solid‐State NMR Spectroscopy
10.5 Nonconventional NMR Techniques
10.5.1 Time Domain NMR
10.5.2 Fast Field Cycling NMR Relaxometry
10.5.3 Earth's Magnetic Field NMR
10.6 Case Studies. 10.6.1 Polymers and Polymer‐Based Composites
10.6.2 Mesoporous Materials
10.6.3 Cultural Heritage
10.6.4 Food
10.6.5 Environmental NMR: Rocks, Soils, Waters, Air
10.6.6 NMR of “Exotic” Nuclei
References
11 X‐Ray Absorption Spectroscopy and X‐Ray Raman Scattering Spectroscopy for Energy Applications
11.1 Introduction
11.2 The X‐Ray Absorption Coefficient and the EXAFS Technique
11.2.1 The EXAFS Equation and the Key Approximations
11.2.1.1 Many‐Body Effects
11.2.1.2 Inelastic Effects
11.2.1.2.1 Extrinsic Processes
11.2.1.2.2 Intrinsic Losses
11.2.2 Multiple Scattering Theory: Basic Information
11.2.3 XANES or Near‐Edge X‐Ray Absorption Fine Structure and Pre‐Edge Region
11.3 EXAFS: Data Analysis Overview
11.4 Experimental Setups
11.4.1 Transmission Geometry
11.4.2 Fluorescence Geometry
11.5 X‐Ray Raman Scattering Spectroscopy
11.5.1 Theoretical Background
11.5.2 Experimental Setup. 11.5.2.1 Instrumentation
11.5.2.2 Data Processing
11.6 Case Studies: Application of XAFS and XRS for Energy Materials
11.6.1 CO Oxidation Reaction: The Au/CeO2 Catalyst
11.6.2 Materials for Solid Oxide Fuel Cells
11.6.3 Oxide‐Ion Conductors: Dopants and Vacancies
11.6.4 Proton‐Conducting Oxides
11.6.5 The Role of Oxygen in Fuel Cell Cathodes
References
12 X‐Ray Photoelectron Spectroscopy
12.1 General Principles
12.2 Instrumental Setup
12.2.1 Vacuum and Ultrahigh Vacuum, UHV
12.2.1.1 Roughing Pumps
12.2.1.2 Turbomolecular Pumps
12.2.1.3 Ion Pumps
12.2.1.4 Titanium Sublimation Pumps
12.2.2 Magnetic Shielding
12.2.3 Sources
12.2.4 Sample Manipulators
12.2.5 Charge Neutralization Systems
12.2.5.1 Electron Guns
12.2.5.2 Ion Guns
12.2.6 Analyzers and Detectors
12.3 Applications
12.3.1 Quantitative Analysis
12.3.2 Qualitative Analysis
12.3.3 Surface Maps
12.3.4 Profiles
12.3.4.1 Depth Profiles
12.3.4.2 Angle‐Resolved Profiles
12.4 Data Analysis
12.4.1 Shift Corrections
12.4.2 Background
12.4.3 Line Shapes
12.4.4 Nonlinear Fitting
12.5 Case Studies
12.5.1 Hydrocarbon Contamination
12.5.2 Energy Loss
12.5.3 Depth Profiles/1
12.5.4 Depth Profiles/2
References
Notes
13 Ultraviolet Photoelectron Spectroscopy – Materials Science Technique
13.1 UPS History and Capabilities
13.2 Theory and Experimental Methodology of UPS. 13.2.1 Physical Principles of UPS
13.2.2 Angle‐Resolved UPS
13.3 UPS Experiment and Factors of Influence
13.3.1 Vacuum System and Pumping
13.3.2 Sample and External Spectral Standard Preparation
13.3.3 Ultraviolet Source
13.3.4 Charge Neutralizer
13.3.5 Staff Requirements
References
14 Transmission Electron Spectroscopy
14.1 Empirical Aspects of Electron–Matter Interaction
14.1.1 Fast Electrons Interaction with a Solid
14.1.2 Electron Energy Loss Spectroscopy (EELS)
14.1.2.1 Inner Shell Excitations
14.1.2.2 Low‐Loss Excitations
14.1.2.3 Energy‐Filtered Images
14.2 Instrumental Setups. 14.2.1 TEM in a Nutshell
References
15 Atomic Force Microscopy and Spectroscopy
15.1 Introduction
15.2 The AFM Microscope
15.2.1 The Probe
15.2.2 Harmonic Excitation of the Cantilever
15.2.3 Scanning System
15.2.4 Measurement of the Cantilever's Deflection
15.2.5 Feedback System
15.3 Tip–Surface Interaction Forces
15.3.1 Van der Waals
15.3.2 Short‐Range Repulsive
15.3.3 Adhesion
15.3.4 Capillary
15.3.5 Other Forces
15.4 AFM Acquisition Modes
15.4.1 Contact Mode
15.4.2 Tapping Mode
15.5 AFM Spectroscopy
15.6 Case Studies
15.6.1 Roughness of a Flat Surface
15.6.2 Size Distribution of Nanoparticles
References
Index
WILEY END USER LICENSE AGREEMENT
Отрывок из книги
Edited by
.....
(1.68)
Introducing now the rate equation for the excited state
.....