Microbial Interactions at Nanobiotechnology Interfaces
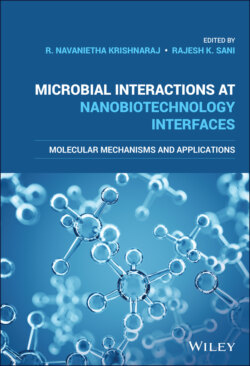
Реклама. ООО «ЛитРес», ИНН: 7719571260.
Оглавление
Группа авторов. Microbial Interactions at Nanobiotechnology Interfaces
Table of Contents
List of Tables
List of Illustrations
Guide
Pages
Microbial Interactions at Nanobiotechnology Interfaces. Molecular Mechanisms and Applications
Preface
List of Contributors
1 Shape‐ and Size‐Dependent Antibacterial Activity of Nanomaterials
Objectives
1.1 Introduction
1.2 Synthesis of Nanomaterials
1.3 Classification of NMs
1.3.1 Classification Based on Dimensions
1.3.1.1 Zero‐Dimensional NMs
1.3.1.2 One‐Dimensional NMs
1.3.1.3 Two‐Dimensional NMs
1.3.1.4 Three‐Dimensional NMs
1.3.2 Classification Based on Chemical Compositions
1.3.2.1 Carbon‐Based NMs
1.3.2.2 Organic‐Based NMs
1.3.2.3 Inorganic‐Based NMs
1.3.2.3.1 Metal‐Based NMs
1.3.2.3.2 Metal Oxide‐Based NMs
1.3.2.3.3 Composite‐Based NMs
1.3.3 Classification Based on Origin
1.4 Application of NMs
1.4.1 Advanced Application of NMs as Antimicrobial Agents
1.5 Bacterial Resistance to Antibiotics
1.5.1 Mechanism of Antibiotic Resistance
1.5.1.1 Antibiotics Modification
1.5.1.2 Antibiotic Efflux
1.5.1.3 Target Modification or Bypass or Protection
1.6 Microbial Resistance: Role of NMs
1.6.1 Overcoming the Existing Antibiotic Resistance Mechanisms
1.6.1.1 Combating Microbes Using Multiple Mechanisms Simultaneously
1.6.1.2 Acting as Good Carriers of Antibiotics
1.7 Antibacterial Application of NMs
1.7.1 Nanometals
1.7.2 Metal Oxides
1.7.3 Carbonaceous NMs
1.7.4 Cationic Polymer NMs
1.8 Interaction of NMs with Bacteria
1.9 Antibacterial Mechanism of NMs
1.10 Factors Affecting the Antibacterial Activity of NMs
1.10.1 Size
1.10.2 Shape
1.10.3 Zeta Potential
1.10.4 Roughness
1.10.5 Synthesis Methods and Stabilizing Agents
1.10.6 Environmental Conditions
1.11 Influence of Size on the Antibacterial Activity and Mechanism of Action of Nanomaterials
1.12 Influence of Shape on the Antibacterial Activity and Mechanism of Action of Nanomaterials
1.13 Effects of Functionalization on the Antimicrobial Property of Nanomaterials
1.14 Conclusion and Future Perspectives
Questions and Answers
References
Keywords
Glossary
2 Size‐ and Shape‐Selective Synthesis of DNA‐Based Nanomaterials and Their Application in Surface‐Enhanced Raman Scattering
Objectives
2.1 Introduction
2.2 Mechanism of Surface‐Enhanced Raman Scattering (SERS)
2.2.1 Significance of Nano‐Bio Interfaces and Role of DNA in Enhancing SERS Activity
2.3 Size‐ and Shape‐Selective Synthesis of Metal NPs with DNA for SERS Studies
2.3.1 Metal NP Assemblies on DNA Using Photochemical Route for SERS Studies
2.3.2 Metal NP Assemblies on DNA Using Chemical Reduction Process as Aquasol for SERS Studies
2.3.3 Metal NP Assemblies on DNA Using Chemical Reduction as Organosol for SERS Studies
2.3.4 Metal NP Assemblies on DNA Prepared Using Microwave Heating for SERS Studies
2.3.5 Conclusions and Outcomes of DNA‐Based Metal Nanostructures for SERS Studies
Take Home Message
Questions and Answers
Acknowledgements
References
Glossary
Academic Profile
3 Surface Modification Strategies to Control the Nanomaterial–Microbe Interplay
Objectives
3.1 Introduction
3.2 Factors Influencing NM–Microbe Cross talk
3.2.1 Surface Features of Microbes
3.2.2 Physicochemical Properties of NMs
3.3 Surface Functionalization
3.3.1 Techniques Used for Surface Functionalization
3.3.1.1 Self‐Assembled Monolayers
3.3.1.2 Layer‐by‐Layer Technique
3.3.2 Surface Functionalization Strategies
3.3.2.1 Physicochemical Modifications
3.3.2.2 Biofunctionalization
3.3.2.2.1 Covalent Immobilization and Bioconjugation‐Based Systems
3.3.2.2.2 Release Systems
3.4 Characterization of NM–Microbe Interactions
3.4.1 Microbe Parameters
3.4.2 NM Parameters
3.5 Toxicity of the Surface‐Modified NMs
3.6 Challenges and Future Perspectives
Questions and Answers
Take Home Message
References
Glossary
4 Surface Functionalization of Nanoparticles for Stability in Biological Systems
Objectives
4.1 Introduction
4.2 Major Processes Affecting NP Stability in Biological Media
4.2.1 Aggregation
4.2.2 Nanoparticle Design and Properties
4.2.3 Hydrophobicity/Hydrophilicity Effects
4.2.4 Effect of Protein Corona
4.2.4.1 Effect of Protein Corona on Active Targeting
4.2.5 External Factors
4.3 Measures to Enhance NP Stability in Biological Systems. 4.3.1 Stabilization Against Aggregation
4.3.2 Ligand Exchange
4.3.3 Coating with Additional Layers
4.3.3.1 Silica Coating
4.3.3.2 PEG Coating
4.3.3.2.1 PEG Coating of Plasmonic NPs. Citrate‐Stabilized Particles
CTAB‐Stabilized Particles
4.3.3.2.2 PEG Coating of Magnetic NPs
4.3.3.2.3 PEG Coating on Quantum Dots (QDs)
4.3.3.3 Lipid Bilayer Coating
4.3.3.3.1 Lipid Bilayer Coating of Plasmonic NPs
4.3.3.3.2 Lipid Bilayer Coating of Magnetic NPs
4.3.3.3.3 Lipid Bilayer Coating of Fluorescent NPs
4.3.3.4 Zwitterionic Coating
4.3.3.4.1 Zwitterionic Coating on Plasmonic NPs
4.3.3.4.2 Zwitterionic Coating on QDs
4.3.3.4.3 Zwitterionic Coating on Magnetic NPs
4.3.3.5 Protein Coating
4.3.3.5.1 Protein Coating on Plasmonic NPs
4.3.3.5.2 Protein Coating on QDs
4.3.3.5.3 Protein Coating on Magnetic NPs
4.3.3.6 Aptamer Coating
4.3.3.6.1 Aptamer Coating on Plasmonic NPs
4.3.3.6.2 Aptamer Coating on QDs
4.3.3.6.3 Aptamer Coating on Magnetic NPs
4.3.4 Subsiding the Nonspecific Protein Interaction
4.3.5 Nanoparticle Design
4.3.5.1 Particle Functionalization
4.3.5.1.1 Chemical Functional Groups (Covalent Coupling)
4.3.5.1.2 Amide Group Involved Coupling
4.3.5.1.3 Thiol Group Coupling
4.3.5.1.4 Click Chemistry
4.3.6 Influence of NM Physicochemical Properties on Microbe–NM Interaction
4.4 Conclusion and Future Perspectives
4.5 Summary
Questions and Answers
References
Glossary
5 Molecular Mechanisms Behind Nano‐Cancer Therapeutics
Objectives
5.1 Nanotechnology at Nano–Bio Interfaces
5.2 Armory of Nanomedicine at Nano–Bio Interfaces
5.3 Nanoparticle Edge in Modulating Biological Process
5.4 Intracellular Uptake and Trafficking of Nanoparticle
5.5 Challenges in Clinical Applications
5.6 Conclusion
Take Home Message
Questions and Answers
Acknowledgements
References
Glossary
6 Protein Nanoparticle Interactions and Factors Influencing These Interactions
Objectives
6.1 Introduction
6.2 Types and Biomedical Application of Nanoparticles
6.3 Methods and Mechanisms of Nanomaterials Synthesis
6.4 Routes of Entry of Nanoparticles into Biological System
6.5 Rationale for Studying Nanoparticles–Protein Interactions
6.6 Formation of Protein Corona. 6.6.1 Structure and Composition of Corona
6.6.2 Kinetics of Formation of Nanoparticles–Corona
6.7 Nanoparticles‐Induced Structural Changes in Proteins. 6.7.1 Reversible
6.7.2 Irreversible
6.8 Factors Influencing Corona Formation
6.8.1 Properties of Nanoparticles
6.8.1.1 Size
6.8.1.2 Shape
6.8.1.3 Charge
6.8.1.4 Surface Functionalization
6.8.1.5 Surface Reactivity
6.8.1.6 Solubility
6.8.2 Properties of Protein
6.8.3 Effect of Surrounding Environment. 6.8.3.1 Effect of Media Composition on Corona Formation
6.8.3.2 Effect of Temperature
6.8.3.3 Static In Vitro Model Vs. Dynamic In Vivo System
6.9 Interaction of Nanoparticles with Cells and Their Uptake
6.10 Pleiotrophic Effect of Nanoparticles
6.11 Analytical Methods to Study Nanoparticles–Protein Interaction. 6.11.1 Spectral Properties. 6.11.1.1 UV–Vis Spectroscopy
6.11.1.2 FTIR
6.11.1.3 Raman Spectroscopy
6.11.1.4 Fluorescence Spectroscopy
6.11.2 Surface Plasmon Resonance
6.11.3 Cellular Uptake of Nanoparticles–Protein. 6.11.3.1 Flow Cytometry
6.11.3.2 Confocal Microscopy
6.11.4 Binding Affinity. 6.11.4.1 Differential Scanning Calorimetry and Isothermal Calorimetry
6.11.4.2 Isothermal Titration Calorimetry
Questions and Answers
References
Glossary
7 Interaction Effects of Nanoparticles with Microorganisms Employed in the Remediation of Nitrogen‐Rich Wastewater
Objectives
7.1 Introduction
7.2 Bacterial Nitrification Process
7.2.1 Effect of NPs on Functional Gene Abundance and Transcriptional Response
7.2.2 Effect of NPs on Enzyme Activity
7.2.3 Effect on Cellular Morphology
7.3 Effect of NPs on Denitrifying Bacteria
7.3.1 Effect on Functional Gene Abundance and Transcriptional Response
7.3.2 Enzymatic Response
7.4 Impact of Nanoparticles on Nitrogen Removal
7.5 Conclusion
Take Home Message
Questions and Answers
Acknowledgements
References
Glossary
8 Silver‐Based Nanoparticles for Antibacterial Activity: Recent Development and Mechanistic Approaches
Objectives
8.1 Introduction
8.2 Historical Background of Silver
8.3 Synthesis Procedures of Silver Nanoparticles
8.3.1 Chemical Synthesis
8.3.2 Physical Methods
8.3.3 Biological Methods
8.4 Biological Application of Silver Nanoparticles
8.5 Bacterial Infection and Antibiotic Resistance
8.6 Nanosilver for Antibacterial Therapy
8.6.1 Metallic Silver Nanoparticles
8.6.2 Biosynthesized Silver Nanoparticles
8.6.3 Silver Nanocomposites
8.6.4 Silver Nanoscaffolds
8.7 Influence of Size and Shape of Silver Nanoparticles as Antibacterial Agents
8.8 Nanosilver and Its Mechanism of Action for Antibacterial Therapy
8.9 Application of Silver Nanoparticle in Commercial Products
8.9.1 Silver Nanoparticles in Wound Dressing Materials and Devices
8.9.2 Silver Nanoparticles in Soaps and Detergents
8.9.3 Silver Nanoparticles in Fabrics
8.9.4 Silver Nanoparticles in Cosmetics
8.9.5 Silver Nanoparticles in Food Packaging
8.9.6 Silver Nanoparticles in Paints
8.10 Toxicity of Silver Nanoparticles
8.11 Future Prospective and Challenges
8.12 Conclusion
Take Home Message
Questions and Answers
Abbreviation
Acknowledgments
References
Glossary
9 Microbial Gold Nanoparticles and Their Biomedical Applications
Objectives
9.1 Introduction
9.2 Microbial Gold Nanoparticles Synthesis
9.2.1 Bacteria‐Mediated Gold Nanoparticles
9.2.2 Algae‐Mediated Gold Nanoparticles
9.2.3 Fungi‐Mediated Gold Nanoparticles
9.2.4 Yeast‐Mediated Gold Nanoparticles
9.2.5 Mechanism Involved in Microbial Nanoparticles Synthesis
9.3 Applications of Microbial Gold Nanoparticles. 9.3.1 Biosensing
9.3.2 Antibacterial Activity of Au NPs
9.3.3 Anticancer Activity of Microbial Gold Nanoparticles
9.4 Conclusion
Acknowledgements
Take Home Message
Questions and Answers
References
Glossary
10 Nano‐Bio Interactions and Their Practical Implications in Agriculture
10.1 Introduction
10.1.1 Agriculturally Beneficial Soil Microorganisms
10.2 Engineered Nanomaterials and Agriculture. 10.2.1 Pathways for ENM to Soil
10.2.2 Fate of ENMs in Soil
10.2.3 Chemical Interactions of ENM in Soil
10.2.4 Mechanisms Controlling Heteroaggregation
10.2.5 Mobility of Colloids and ENMs in Soil
10.2.6 Nanoagriculture
10.2.7 Nanopesticides
10.2.8 ENMs and Agriculturally Beneficial Microorganisms
10.3 Summary
References
11 Biogeochemical Interactions of Bioreduced Uranium Nanoparticles
11.1 Introduction
11.2 Coupled Biogeochemical Mechanisms and Interactions of U in the Subsurface
11.3 Biogenic Uraninite Precipitation and Its Nanoparticulate Forms
11.4 Re‐oxidation and Stability of Bioreduced Uranium
11.5 Summary and Conclusions
Questions and Answers
References
Note
12 Characterization and Quantification of Mobile Bioreduced Uranium Phases
12.1 Introduction
12.2 Characterization of Biogenic U(IV)
12.3 Quantification of Mobile Bioreduced U(IV) Nanoparticles
12.4 Summary and Conclusions
Questions and Answers
References
Note
Index. a
b
c
d
e
f
g
h
i
l
m
n
o
p
q
r
s
t
u
v
w
y
z
WILEY END USER LICENSE AGREEMENT
Отрывок из книги
Edited by
.....
As discussed earlier, the activity of any NM system depends on its physicochemical properties such as size, shape, zeta potential, crystal structure, charge, and other factors. The physicochemical factors' influence on the surface area, surface energy, and atomic ligand deficiency dictates the behavior of NMs, which in turn affects the activity of NMs. Hence it is very important to study the effect of these factors on the activity of the NMs. Schematic of the factors influencing the antimicrobial activity of the NMs is given the Figure 1.5 as detailed by Daima and Bansal (2015).
A key advantage of any NM system is its higher surface area‐to‐volume ratio in comparison to the micro and macro structures. Practically it is possible to contain a significantly high number of smaller NMs in comparison to bigger particles in the same volume. High surface area‐to‐volume ratio owing to an increased number of particles results in exposure of greater numbers of atoms, which could increase the activity. Considering the case of antibacterial system, the formation of biofilm is a key event in the development of resistant bacteria. Exposure of a greater number of atoms on the surface results in increased interaction of NM surface with bacteria, increasing the number of reactive oxygen species at a faster rate followed by inhibition or elimination of the bacteria. Supporting this fact, several recent studies have shown that the size of NMs plays a critical role in dictating the antimicrobial property of the NM.
.....