Polymer Composites for Electrical Engineering
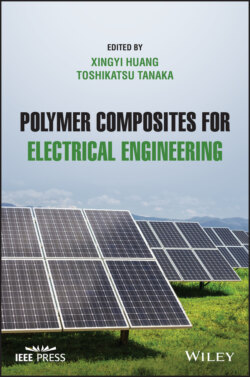
Реклама. ООО «ЛитРес», ИНН: 7719571260.
Оглавление
Группа авторов. Polymer Composites for Electrical Engineering
Table of Contents
List of Tables
List of Illustrations
Guide
Pages
Polymer Composites for Electrical Engineering
List of Contributors
Preface
1 Polymer Composites for Electrical Energy Storage
1.1 Introduction
1.2 General Considerations
1.3 Effect of Nanofiller Dimension
1.4 Orientation of Nanofillers
1.5 Surface Modification of Nanofillers
1.6 Polymer Composites with Multiple Nanofillers
1.7 Multilayer‐structured Polymer Composites
1.8 Conclusion
References
2 Polymer Composites for Thermal Energy Storage
2.1 Introduction
2.2 Shape‐stabilized Polymeric Phase Change Composites
2.2.1 Micro/Nanoencapsulated Method
2.2.2 Physical Blending
2.2.3 Porous Supporting Scaffolds
2.2.4 Solid–Solid Composite PCMs
2.3 Thermally Conductive Polymeric Phase Change Composites
2.3.1 Metals
2.3.2 Carbon Materials
2.3.3 Ceramics
2.4 Energy Conversion and Storage Based on Polymeric Phase Change Composites
2.4.1 Electro‐to‐Heat Conversion
2.4.2 Light‐to‐Heat Conversion
2.4.3 Magnetism‐to‐Heat Conversion
2.4.4 Heat‐to‐Electricity Conversion
2.5 Emerging Applications of Polymeric Phase Change Composites
2.5.1 Thermal Management of Electronics
2.5.2 Smart Textiles
2.5.3 Shape Memory Devices
2.6 Conclusions and Outlook
Acknowledgments
References
3 Polymer Composites for High‐Temperature Applications
3.1 Applications of Polymer Composite Materials in High‐Temperature Electrical Insulation
3.1.1 High‐Temperature‐Resistant Electrical Insulating Resin Matrix
3.1.1.1 Silicone Resins
3.1.1.2 Polyimide
3.1.1.3 Polyether Ether Ketone
3.1.1.4 Polybenzimidazole
3.1.1.5 Polyphenylquinoxaline
3.1.1.6 Benzoxazine
3.1.2 Modification of Resin Matrix with Reinforcements
3.1.2.1 Mica
3.1.2.2 Glass Fiber
3.1.2.3 Inorganic Nanoparticles
3.1.3 Modifications in the Thermal Conductivity of Resin Matrix
3.1.3.1 Mechanism of Thermal Conductivity
3.1.3.2 Intrinsic High Thermal Conductivity Insulating Material
3.1.3.3 Filled High Thermal Conductivity Insulating Material
3.2 High‐Temperature Applications for Electrical Energy Storage
3.2.1 General Considerations for High‐Temperature Dielectrics
3.2.2 High‐Temperature‐Resistant Polymer Matrix
3.2.3 Polymer Composites for High‐Temperature Energy Storage Applications
3.2.4 Surface Modification of Nanocomposite forHigh‐Temperature Applications
3.2.5 Sandwich Structure of Nanoparticles forHigh‐Temperature Applications
3.3 Applications of High‐Temperature Polymer in Electronic Packaging
3.3.1 Synthesis of Low Dielectric Constant Polymer Materials Through Molecular Structure Design. 3.3.1.1 Fluorine‐Containing Low Dielectric Constant Polymer
3.3.1.2 Low Dielectric Constant Polymer Material Containing NonpolarRigid Bulk Group
3.3.2 High‐Temperature‐Resistant Low Dielectric Constant Polymer Composite Material
3.3.2.1 Low Dielectric Constant Polyoxometalates/Polymer Composite
3.3.2.2 Low Dielectric Constant POSS/Polymer Composite
3.4 Applications of Polymer Composite Materials in the Field of High‐Temperature Wave‐Transmitting and Wave‐Absorbing Electrical Fields
3.4.1 Wave‐Transmitting Materials
3.4.1.1 The High‐Temperature Resin Matrix
3.4.1.2 Reinforced Materials
3.4.2 Absorbing Material
3.4.2.1 The High‐Temperature Resin Matrix
3.4.2.2 Inorganic Filler
3.5 Summary
References
4 Fire‐Retardant Polymer Composites forElectrical Engineering
4.1 Introduction
4.2 Fire‐Retardant Cables and Wires. 4.2.1 Fundamental Overview
4.2.2 Understanding of Fire‐Retardant Cables and Wires. 4.2.2.1 Polyethylene Composites
4.2.2.2 Ethylene‐Vinyl Acetate (EVA) Copolymer
4.2.2.3 Polyvinyl Chloride Composites
4.2.2.4 Other Polymers
4.3 Fire‐Retardant Polymer Composites for Electrical Equipment. 4.3.1 Fundamental Overview
4.3.2 Understanding of Fire‐Retardant Polymer Composites for Electrical Equipment. 4.3.2.1 HIPS and ABS Composites
4.3.2.2 PC/ABS Composites
4.3.2.3 PC Composites
4.3.2.4 PBT Composites
4.4 Fire‐Retardant Fiber Reinforced Polymer Composites. 4.4.1 Fundamental Overview
4.4.2 Understanding of Fire‐Retardant Fiber Reinforced Polymer Composites. 4.4.2.1 Reinforced PBT and PET Composites
4.5 Conclusion and Outlook
References
5 Polymer Composites for Power Cable Insulation
5.1 Introduction
5.2 Trend in Nanocomposite Materials for Cable Insulation
5.2.1 Overview
5.2.2 Polymer Materials as Matrix Resin
5.2.3 Fillers
5.2.4 Nanocomposites
5.2.4.1 XLPE Nanocomposites
5.2.4.2 PP Nanocomposites
5.2.4.3 Nanocomposite with Cluster/Cage Molecule
5.2.4.4 Copolymer and Polymer Blend
5.3 Factors Influencing Properties
5.4 Issues in Nanocomposite Insulation Materials Research
5.5 Understanding Dielectric and Insulation Phenomena
5.5.1 Electromagnetic Understanding
5.5.2 Understanding Space Charge Behavior by Q(t) Method
References
6 Semi‐conductive Polymer Composites for Power Cables
6.1 Introduction
6.1.1 Function of Semi‐conductive Composites
6.1.2 Development of Semi‐conductive Composites
6.2 Conductive Mechanism of Semi‐conductive Polymer Composites
6.2.1 Percolation Theory
6.2.2 Tunneling Conduction Theory
6.2.3 Mechanism of Positive Temperature Coefficient
6.3 Effect of Polymer Matrix on Semi‐conductivity
6.3.1 Thermoset Polymer Matrix
6.3.2 Thermoplastic Polymer Matrix
6.3.3 Blended Polymer Matrix
6.4 Effect of Conductive Fillers on Semi‐conductivity
6.4.1 Carbon Black
6.4.2 Carbonaceous Fillers with One‐ and Two‐Dimensions
6.4.3 Secondary Filler for Carbon Black Filled Composites
6.5 Effect of Semi‐conductive Composites on Space Charge Injection
6.6 Conclusions
References
7 Polymer Composites for Electric Stress Control
7.1 Introduction
7.2 Functionally Graded Solid Insulators and Their Effect on Reducing Electric Field Stress
7.3 Practical Application of ε‐FGMs to GIS Spacer
7.4 Application to Power Apparatus
References
8 Composite Materials Used in Outdoor Insulation
8.1 Introduction
8.2 Overview of SIR Materials
8.2.1 RTV Coatings
8.2.2 Composite Insulators
8.2.3 Liquid Silicone Rubber (LSR)
8.2.4 Aging Mechanism and Condition Assessment of SIR Materials
8.3 New External Insulation Materials
8.3.1 Anti‐icing Semiconductor Materials
8.3.2 Hydrophobic CEP
8.4 Summary
References
9 Polymer Composites for Embedded Capacitors
9.1 Introduction. 9.1.1 Development of Embedded Technology
9.1.2 Dielectric Materials for Commercial Embedded Capacitors
9.2 Researches on the Polymer‐Based Dielectric Nanocomposites
9.2.1 Filler Particles
9.2.2 Epoxy Matrix
9.2.2.1 Modification to Improve Dielectric Properties
9.2.2.2 Modification to Improve Mechanical Properties
9.3 Fabrication Process of Embedded Capacitors
9.4 Reliability Test of Embedded Capacitor Materials
9.5 Conclusions and Perspectives
References
10 Polymer Composites for Generators and Motors
10.1 Introduction
10.2 Polymer Composite in High‐Voltage Rotating Machines
10.3 Ground Wall Insulation. 10.3.1 Mica/Epoxy Insulation
10.3.2 Electrical Defect in the Insulation of Rotating Machines and Degradation Mechanism
10.3.3 Insulation Design and V‐t Curve
10.4 Polymer Nanocomposite for Rotating Machine
10.4.1 Partial Discharge Resistance and a Treeing Lifetime of Nanocomposite as Material Property. 10.4.1.1 PD Resistance
10.4.1.2 Electrical Treeing Lifetime
10.4.2 Breakdown Lifetime Properties of Realistic Insulation Defect in Rotating Machine
10.4.2.1 Voltage Endurance Test of Void Defect
10.4.2.2 Voltage Endurance Test in Mica/Epoxy Nanocomposite‐Layered Structure
10.4.2.3 V‐t Curves in Coil Bar Model with Mica/Epoxy Nanocomposite Insulation
10.5 Stress‐Grading System of Rotating Machines. 10.5.1 Silicon Carbide Particle‐Loaded Nonlinear‐Resistive Materials
10.5.2 End‐turn Stress‐Grading System of High‐Voltage Rotating Machines
References
11 Polymer Composite Conductors and Lightning Damage
11.1 Lightning Environment and Lightning Damage Threat to Composite‐Based Aircraft. 11.1.1 The Lightning Environment. 11.1.1.1 Formation of Lightning
11.1.2 Lightning Test Environment of Aircrafts
11.1.2.1 Zone 1
11.1.2.2 Zone 2
11.1.2.3 Zone 3
11.1.2.4 Current Component A – First Return Strike
11.1.2.5 Current Component Ah – Transition Zone First Return Strike
11.1.2.6 Current Component B – Intermediate Current
11.1.2.7 Current Component C – Continuing Current
11.1.2.8 Component C* – Modified Component C
11.1.2.9 Current Component D – Subsequent Strike Current
11.1.3 Waveform Combination in Different Lightning Zones for Lightning Direct Effect Testing
11.1.4 Application of CFRP Composites in Aircraft
11.2 The Dynamic Conductive Characteristics of CFRP. 11.2.1 A Review of the Research on the Conductivity of CFRP
11.2.2 The Testing Methods
11.2.2.1 Specimens
11.2.2.2 The Test Fixture
11.2.2.3 Lightning Impulse Generator and Lightning Waveforms
11.2.3 The Experimental Results of the Dynamic Impedance of CFRP. 11.2.3.1 The Nondestructive Lightning Current Test
11.2.3.2 The Applied Lightning Current Impulse and the Response Voltage Impulse
11.2.3.3 Equivalent Conductivity of CFRP Laminates Under Different Lightning Impulses
11.2.3.4 Equivalent Conductivity of CFRP Laminates with Different Laminated Structures
11.2.4 The Discussion of the Dynamic Conductive Characteristics of CFRP. 11.2.4.1 The Conduction Path of the CFRP Laminate Under a Lightning Current Impulse
11.2.4.2 Dynamic Conductance of CFRP Laminate
11.2.4.3 The Inductive Properties of CFRP Laminates
11.2.4.4 Equivalent Conductivity of CFRP Laminates Subjected to Lightning Current Impulses with Higher Intensity
11.3 The Lightning Strike‐Induced Damage of CFRP Strike. 11.3.1 Introduction of the Lightning Damage of CFRP
11.3.2 Single Lightning Strike‐Induced Damage. 11.3.2.1 Experimental Setup for Single Lightning Strike Test
11.3.2.2 Experimental Results of Single Lightning Strike‐Induced Damage. 11.3.2.2.1 Lightning Strike Process
11.3.2.2.2 Surface Damage of the CFRP Laminates
11.3.2.2.2.1 Zone I
11.3.2.2.2.2 Zone II
11.3.2.2.2.3 Zone III
11.3.2.2.3 Internal Damage Behavior
11.3.2.3 Evaluation for Single Lightning Strike‐Induced Damage
11.3.3 Multiple Lightning Strikes‐Induced Damage
11.3.3.1 Experimental Method for Multiple Consecutive Lightning Strike Tests. 11.3.3.1.1 Test Specimens
11.3.3.1.2 Experimental Setup
11.3.3.1.3 Test Method for Multiple Lightning Damage Test
11.3.3.2 Experimental Results of Multiple Lightning Damage. 11.3.3.2.1 Visual Inspection of Lightning Damage
11.3.3.2.2 Temperature Properties of CFRP Laminates After Multiple Lightning Strike
11.3.3.2.3 Internal Damage Images
11.3.3.3 Multiple Lightning Damage Areas and Depths of CFRP Laminates
11.3.3.4 Analysis for Multiple Lightning Damage of CFRP Laminates
11.3.3.4.1 Lightning Damage Depth
11.3.3.4.2 Lightning Damage Area
11.3.3.4.3 Comparison of the Lightning Damage of Different Test Modes of Lightning Strike. 11.3.3.4.3.1
11.3.3.4.3.2 The Comparison of Lightning Damage Effect in “ABC” and “DBC” Test Modes
11.3.3.4.3.3 The Influence of Material Properties on Lightning Damage of Multiple Sequential Lightning Strikes
11.3.3.5 Evaluation for Multiple Lightning Damage of CFRP Laminates. 11.3.3.5.1 Evaluation for the Lightning Damage Depth
11.3.3.5.1.1 Influence of the Current Amplitude on the Lightning Damage Depth
11.3.3.5.1.2 Influence of the Rise Rate on the Lightning Damage Depth
11.3.3.5.1.3 Evaluation for the Lightning Damage Area
11.3.3.5.1.4 The Influence of the Electrical Action Integral on the Lightning Damage Area
11.3.3.5.1.5 Influence of the Transfer Charge on the Lightning Damage Area
11.3.3.5.2 Summary of the Evaluation Methods
11.4 The Simulation of Lightning Strike‐Induced Damage of CFRP. 11.4.1 Overview of Lightning Damage Simulation Researches
11.4.2 Establishment of the Coupled Thermal‐Electrical Model. 11.4.2.1 Finite Element Model
11.4.2.2 Simulated Lightning Component A
11.4.2.3 Pyrolysis Degree Calculation
11.4.2.4 Dynamic Conductive Properties
11.4.2.5 Pyrolysis‐Dependent Material Parameters
11.4.3 Simulation Physical Fields of Lightning Current on CFRP Laminates. 11.4.3.1 Temperature and Pyrolysis Fields
11.4.3.2 Mechanical Analysis
11.4.4 Simulated Lightning Damage Results. 11.4.4.1 Numerical Criterion for Lightning Damage
11.4.4.1.1 Temperature Field Criterion
11.4.4.1.2 Pyrolysis Degree Field Criterion
11.4.4.2 In‐Plane Lightning Damage Evaluation
11.4.4.3 In‐Depth Lightning Damage Evaluation
References
12 Polymer Composites for Switchgears
12.1 Introduction
12.2 History of Switchgear
12.3 Typical Insulators in Switchgears. 12.3.1 Epoxy‐based Composite Insulators
12.3.2 Insulator‐Manufacturing Process
12.3.2.1 Vacuum Casting Method
12.3.2.2 Automatic Pressure Gelation Method
12.3.2.3 Vacuum Pressure Impregnation Method
12.4 Materials for Epoxy‐based Composites
12.4.1 Epoxy Resins
12.4.2 Hardeners
12.4.3 Inorganic Fillers and Fibers
12.4.4 Silane Coupling Agents
12.4.5 Fabrication of Epoxy‐based Composites
12.5 Properties of Epoxy‐based Composites
12.5.1 Necessary Properties of Epoxy‐based Composites for Switchgears
12.5.2 Resistance to Thermal Stresses. 12.5.2.1 Glass Transition Temperature
12.5.2.2 Coefficient of Thermal Expansion (CTE)
12.5.3 Resistances to Electrical Stresses. 12.5.3.1 Short‐term Insulation Breakdown
12.5.3.2 Long‐term Insulation Breakdown (V‐t Characteristics)
12.5.3.3 Relative Permittivity and Resistivity
12.5.4 Resistances to Ambient Stresses. 12.5.4.1 Resistance to SF6 Decomposition Gas
12.5.4.2 Water Absorption
12.5.5 Resistances to Mechanical Stresses. 12.5.5.1 Flexural and Tensile Strength
12.5.5.2 Creep
12.5.6 International Standards for Evaluation of Composites
12.6 Advances of Epoxy‐based Composites for Switchgear
12.6.1 Nanocomposites
12.6.2 High Thermal Conductive Composites
12.6.3 Biomass Material‐Based Composites
12.6.4 Functionally Graded Materials
12.6.5 Estimate of Remaining Life of Composites
12.7 Conclusion
References
13 Glass Fiber‐Reinforced Polymer Compositesfor Power Equipment
13.1 Overview
13.2 Glass Fiber‐Reinforced Polymer Composites. 13.2.1 Fibers
13.2.1.1 Chemical Description
13.2.1.2 Classification of Glass Fibers
13.2.1.3 Properties of Glass Fiber
13.2.1.4 Glass Fabrics
13.2.1.5 Advantages and Disadvantages
13.2.1.6 Common Manufacturing Methods
13.2.1.7 Applications of Glass Fiber in Various Industries
13.2.2 Polymers
13.2.2.1 Epoxy
13.2.2.2 Polyester (Thermosetting)
13.2.2.3 Phenolic
13.2.3 Manufacturing Methods
13.2.4 Specifications of Several Kinds of GFRP Materials. 13.2.4.1 Rigid Laminated Sheets
13.2.4.2 Industrial Rigid Round Laminated Rolled Tubes (Tables 13.10 and 13.11)
13.2.4.3 Insulated Pipe (Table 13.12)
13.2.4.4 Insulated Pull Rod
13.3 Application of Glass Fiber‐Reinforced Polymer Composites. 13.3.1 Laminated Sheets
13.3.2 Composite Long Rod Insulators
13.3.3 UHV‐Insulated Pull Rod for GIS
13.3.4 Composite Pole
13.3.5 Aluminum Conductor Composite Core in an Overhead Conductor
13.3.6 Composite Station Post Insulators
13.3.7 Composite Hollow Insulators
13.3.8 Composite Crossarms
References
Index
a
b
c
d
e
f
g
h
i
k
l
m
n
o
p
r
s
t
u
v
w
x
z
WILEY END USER LICENSE AGREEMENT
Отрывок из книги
Edited by
.....
Figure 1.11 (a) Schematic of the trilayer‐structured film composed of PVDF/BNNS as outer layers and PVDF/BST as the middle layer, (b) cross‐sectional SEM image of trilayer‐structured polymer composites, (c) discharged energy density, and (d) charge/discharge efficiency of PVDF‐based composites with various compositions and structures.
Source: Liu et al. [87]. Reproduced with permission of John Wiley & Sons.
.....