Superatoms
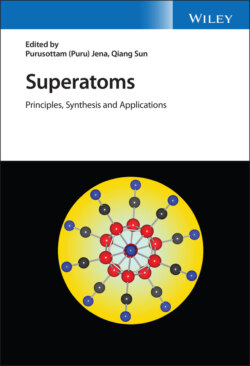
Реклама. ООО «ЛитРес», ИНН: 7719571260.
Оглавление
Группа авторов. Superatoms
Table of Contents
List of Tables
List of Illustrations
Guide
Pages
Superatoms. Principles, Synthesis and Applications
Preface
List of Contributors
1 Introduction
References
2 Rational Design of Superatoms Using Electron‐Counting Rules
2.1 Introduction
2.2 Electron‐Counting Rules. 2.2.1 Jellium Rule
2.2.2 Octet Rule
2.2.2.1 Superalkalis and Superhalogens
2.2.2.2 Superchalcogens
2.2.3 18‐Electron Rule
2.2.4 32‐Electron Rule
2.2.5 Aromaticity Rule
2.2.6 Wade‐Mingos Rule
2.3 Stabilizing Negative Ions Using Multiple Electron‐Counting Rules
2.3.1 Monoanions
2.3.2 Dianions
2.3.3 Trianions
2.3.4 Tetra‐Anions and Beyond
2.4 Conclusions
References
3 Superhalogens – Enormously Strong Electron Acceptors
3.1 Superhalogen Concept. 3.1.1 Early Studies
3.1.2 Further Research (until 1999)
3.1.3 First Measurement of Gas‐Phase Experimental Electron Detachment Energies
3.1.4 The Performance of Theoretical Treatments in Estimating VDEs
3.2 Alternative Superhalogens
3.2.1 Nonmetal Central Atoms
3.2.2 Nonhalogen Ligands
3.2.3 Beyond the MXk + 1 Formula
3.2.4 Superhalogens as Ligands
3.3 Polynuclear Systems and the Search for EA and VDE Limits
3.3.1 Polynuclear Superhalogens
3.3.2 Search for EA and VDE Limits
3.3.3 Magnetic Superhalogens
3.4 Superhalogens' Applications at a Glance
3.5 Final Remarks
Acknowledgements
References
4 Endohedrally Doped Superatoms and Assemblies
4.1 Introduction
4.2 Magic Clusters and Their Electronic Stability
4.3 Discovery of Silicon Fullerenes and Other Polyhedral Forms
4.4 Endohedral Superatoms of Ge, Sn, and Pb
4.5 Magnetic Superatoms
4.6 Endohedral Clusters of Group 11 Elements
4.7 Endohedral Clusters of B, Al, and Ga
4.8 Hydrogenated Silicon Fullerenes
4.9 Compound Superatoms and Other Systems
4.10 Assemblies of Superatoms
4.11 Concluding Remarks
Acknowledgements
References
5 Magnetic Superatoms
5.1 Introduction
5.2 The Arrival of the Magnetic Superatom
5.3 Tunable Superatoms
5.4 The Delocalisation of d‐electrons
5.5 Prospects for Nanostructured Magnetic Material Design
References
6 Atomically Precise Synthesis of Chemically Modified Superatoms
6.1 Introduction. 6.1.1 The Concept of Superatoms
6.1.2 Chemically Modified Au/Ag Superatoms
6.2 Electronic Structures of Chemically Modified Superatoms. 6.2.1 Size Effects
6.2.2 Composition Effects
6.2.3 Shape Effects
6.3 Atomically Precise Synthesis of Chemically Modified Superatoms. 6.3.1 Size Control
6.3.1.1 Top‐down Approach: Size Focusing
6.3.1.2 Bottom‐up Approach: Size Convergence
6.3.1.3 Template Method
6.3.1.4 Kinetic Control
6.3.2 Composition Control. 6.3.2.1 Co‐reduction Method
6.3.2.2 Antigalvanic Method
6.3.2.3 Hydride‐Mediated Transformation
6.3.3 Shape Control
6.3.4 Surface Control
6.3.4.1 Ligand Exchange
6.3.4.2 Hydrogen‐Mediated Transformation
6.4 Summary
References
7 Atomically Precise Noble Metals in the Nanoscale, Stabilized by Ligands
7.1 Introduction
7.2 Fundamentals. 7.2.1 Free Electron Model and the Kubo Gap
7.2.2 Electron Shell Structure
7.2.3 Ligand‐Stabilized Metal Clusters as Superatoms
7.2.3.1 Case Study: The (Ag44(SR)30)4− Superatom
7.2.4 Transition from Electronic to Atomic Shells
7.3 Applications. 7.3.1 Catalysis
7.3.2 Biological and Medical Applications
7.3.2.1 Case Study: Imaging of Enteroviruses
7.3.3 Self‐Assembling Cluster Materials from Superatoms
7.3.3.1 Case Study: Polymeric 1D Cluster Materials
7.4 Summary and Outlook
References
8 Superatoms as Building Blocks of 2D Materials
8.1 Introduction
8.2 Fullerene‐assembled 2D Materials. 8.2.1 C60‐assembled Monolayer
8.2.1.1 Freestanding vdW C60 Monolayer
8.2.1.2 Freestanding Covalent Polymerized C60 Monolayer
8.2.2 Cn (n = 20, 26, 32, 36)‐assembled Monolayers
8.2.3 Fullerene Monolayers on Substrates
8.3 Si‐based Cluster Assembled 2D Materials
8.3.1 V@Si12 Assembled 2D Monolayer
8.3.1.1 Structure and Stability
8.3.1.2 Electronic and Ferromagnetic Properties
8.3.2 Other TM@Si12 Assembled 2D Monolayers
8.3.3 Ta@Si16 Assembled 2D Monolayer and That on Substrate
8.4 Binary Semiconductor Cluster Assembled 2D Materials
8.4.1 Cd6Se6 Assembled Sheets
8.4.2 X12Y12 Cage Cluster Assembled Monolayer
8.5 Simple and Noble Metal Cluster‐assembled 2D Materials
8.5.1 Mg7 Assembled Monolayer
8.5.2 Au9 and Pt9 Assembled Square Monolayer
8.6 Zintl‐ion Cluster‐assembled 2D Materials
8.6.1 Ge9 Ion Cluster Monolayer
8.6.2 Ti@Au12 Ion Cluster Monolayer
8.7 Chevrel Cluster‐Assembled 2D Materials
8.7.1 Re6Se8 Cluster‐based Monolayer
8.7.2 Co6Se8 Cluster‐based Monolayer
8.8 Summary and Future Perspectives
References
9 Superatom‐Based Ferroelectrics
9.1 Introduction
9.2 Organic Ferroelectrics
9.3 Hybrid Organic‐Inorganic Perovskites
9.4 Supersalts
9.5 Conclusion
References
10 Cluster‐based Materials for Energy Harvesting and Storage
10.1 Introduction
10.2 Cluster‐based Materials for Moisture‐resistant Hybrid Perovskite Solar Cells
10.3 Cluster‐based Materials for Optoelectronic Devices
10.4 Cluster‐based Materials for Solid‐state Electrolytes in Li‐ and Na‐ion Batteries
10.4.1 Halogen‐free Electrolytes
10.4.2 Cluster‐based Antiperovskites for Electrolytes in Li‐ion Batteries
10.4.3 Cluster‐based Antiperovskites for Electrolytes in Na‐ion Batteries
10.5 Cluster‐based Materials for Hydrogen Storage
10.5.1 Hydrogen Interaction Mechanism
10.5.2 Intermediate States
10.5.3 Catalysts for Lowering the Dehydrogenation Temperature
10.6 Clusters Promoting Unusual Reactions
10.6.1 Zn in +III Oxidation State
10.6.2 Covalent Binding of Noble Gas Atoms
10.7 Conclusions
References
11 Thermal and Thermoelectric Properties of Cluster‐based Materials
11.1 Introduction
11.2 Basic Theory. 11.2.1 Thermoelectric Effect
11.2.2 Material Performance
11.2.3 Tuning ZT by Carrier Concentration
11.2.4 Tuning ZT by Electronic Structure
11.2.4.1 Carrier Effective Mass, m*
11.2.4.2 Carrier Mobility
11.3 Low Lattice Thermal Conductivity of Cluster‐based Materials
11.3.1 Crystal Complexity of Cluster‐based Materials
11.3.2 Chemical Bond Hierarchy in Cluster‐based Materials
11.3.3 Structural Disorder in Cluster‐based Materials
11.3.4 Orientational Disorder in Cluster‐based Materials
11.3.4.1 Co6E8(PEt3)6 and [Co6E8(PEt3)6][C60]2
11.3.4.2 Fullerene Assembled Films
11.4 Thermoelectric Properties of some Selected Cluster‐based Materials. 11.4.1 Mo6 and Mo9 Cluster‐based Selenides
11.4.1.1 Crystal Structures
11.4.1.2 Electronic Structures
11.4.1.3 Thermal Properties
11.4.1.4 Thermoelectric Figure of Merit ZT
11.4.2 Boron‐based Cluster Materials
11.4.2.1 Crystal Structures
11.4.2.2 Thermoelectric Properties
11.4.3 Silver‐based Cluster Materials
11.5 Conclusion
References
12 Clusters for CO2 Activation and Conversion
12.1 Introduction
12.2 Superalkali Catalysts
12.2.1 Li‐based Superalkalis for CO2 Activation
12.2.2 Supported or Embedded Superalkalis for CO2 Capture
12.3 Al‐based Clusters for CO2 Capture
12.4 Ligand‐protected Au25 Clusters for CO2 Conversion
12.5 M@Ag24 Clusters for CO2 Conversion
12.6 Cu‐based Clusters for CO2 Conversion
12.7 Metal Encapsulated Silicon Nanocages for CO2 Conversion
12.8 Summary and Perspectives
References
13 Conclusions and Future Outlook
Index. a
b
c
d
e
f
g
h
j
k
l
m
n
o
p
r
s
t
v
w
z
WILEY END USER LICENSE AGREEMENT
Отрывок из книги
Edited by
Purusottam (Puru) Jena
.....
Beijing, China
Haoming Shen School of Materials Science and Engineering, Peking University, Beijing, China Center for Applied Physics and Technology, Peking University, Beijing, China
.....