Distributed Acoustic Sensing in Geophysics
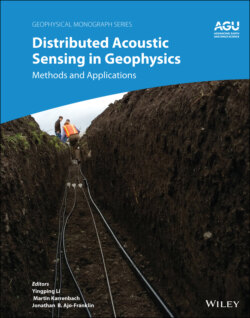
Реклама. ООО «ЛитРес», ИНН: 7719571260.
Оглавление
Группа авторов. Distributed Acoustic Sensing in Geophysics
Table of Contents
List of Tables
List of Illustrations
Guide
Pages
Geophysical Monograph Series
Geophysical Monograph 268
Distributed Acoustic Sensing in Geophysics. Methods and Applications
LIST OF CONTRIBUTORS
LIST OF REVIEWERS
PREFACE
1 High Definition Seismic and Microseismic Data Acquisition Using Distributed and Engineered Fiber Optic Acoustic Sensors
ABSTRACT
1.1. DISTRIBUTED ACOUSTIC SENSOR (DAS) PRINCIPLES AND MEASUREMENTS
1.1.1. DAS Concept
1.1.2. DAS Interferometric Optical Response
1.1.3. DAS Optical Phase Recovery
1.1.4. DAS Dynamic Range Algorithms
1.1.5. DAS Signal Processing and Denoising
1.1.6. Time Integration of DAS Signal
1.2. DAS SYSTEM PARAMETERS AND COMPARISON WITH GEOPHONES
1.2.1. DAS Optimization for Seismic Applications
1.2.2. DAS Directionality in Seismic Measurements
1.2.3. DAS Field Data Examples
1.3. DAS WITH PRECISION ENGINEERED FIBER
1.3.1. Precision engineered fiber concept
1.3.2. Sensitivity and Dynamic Range
1.3.3. Field Trial Results
ACKNOWLEDGMENTS
REFERENCES
TABLE OF VARIABLES
2 Important Aspects of Acquiring Distributed Acoustic Sensing (DAS) Data for Geoscientists
ABSTRACT
2.1. INTRODUCTION
2.2. FIBER‐OPTIC SENSOR. 2.2.1. Sensing from Backscattered Light
2.2.2. Single vs. Multi‐mode Fiber
2.2.3. Deploying Fiber
2.2.4. Handling Fiber‐Optic Cables
2.3. INTERROGATOR UNIT. 2.3.1. Types of Interrogators
2.3.2. Synchronizing Source Information and Time Stamps
2.4. ACQUISITION PARAMETER SELECTION. 2.4.1. Gauge Length
2.4.2. Sampling Rate
2.4.3 Pulse Width
2.5. PREPROCESSING ISSUES. 2.5.1. Fading
2.5.2. Common‐Mode Noise
2.5.3. Spatial Calibration of Channels
2.6. PROCESSING ISSUES. 2.6.1. Angle of Incidence
2.6.2. Single Component vs. Three Components
2.7. DATA QUALITY: DAS VS. GEOPHONE COMPARISONS. 2.7.1. Lower Intrinsic SNR and Higher Channel Density
2.7.2. Strain, Strain Rate, and Particle Velocity
2.8. SUMMARY
REFERENCES
Chapter 3 Distributed Microstructured Optical Fiber (DMOF) Based Ultrahigh Sensitive Distributed Acoustic Sensing (DAS) for Borehole Seismic Surveys
ABSTRACT
3.1. INTRODUCTION
3.2. PRINCIPLES AND METHODS OF DMOF‐DAS. 3.2.1. Principles of DAS Using Optical Fiber
3.2.2. Concept and Characteristics of DMOF
3.2.3. Fabrication and Performance Test of DMOF
3.2.4. System Configuration and Working Principle of the DMOF‐DAS
3.2.5. Performance of the DMOF‐DAS
3.3. BOREHOLE SEISMIC SURVEY TESTS AND RESULTS. 3.3.1. Zero‐Offset VSP Survey in Fushan Oil Field
3.3.2. Walkaway VSP Survey in Suning Oil Field
3.4. DISCUSSIONS
3.5. CONCLUSIONS
ACKNOWLEDGMENTS
REFERENCES
4 Distributed Acoustic Sensing System Based on Phase‐Generated Carrier Demodulation Algorithm
ABSTRACT
4.1. INTRODUCTION
4.2. PRINCIPLE
4.3. EXPERIMENTS AND RESULTS
4.4. FIELD TRIAL OF NEAR‐SURFACE SEISMIC EXPERIMENT WITH PGC‐DAS SYSTEM
4.5. CONCLUSIONS
ACKNOWLEDGMENTS
REFERENCES
5 Field Trial of Distributed Acoustic Sensing in an Active Room‐and‐Pillar Mine
ABSTRACT
5.1. INTRODUCTION
5.2. EXPERIMENTAL METHODS. 5.2.1. Cable Layout and Source Locations
5.2.2. Data Acquisition
5.3. CABLE COUPLING COMPARISONS
5.4. DAS SENSITIVITY
5.5. LOCATING A SEISMIC SOURCE
5.6. SURFACE WAVE TRAVEL‐TIME TOMOGRAPHY
5.7. P‐WAVE DIFFERENTIAL TRAVEL‐TIME TOMOGRAPHY
5.8. DISCUSSION AND CONCLUSIONS
ACKNOWLEDGMENTS
REFERENCES
6 On the Surmountable Limitations of Distributed Acoustic Sensing (DAS) Vertical Seismic Profiling (VSP) – Depth Calibration, Directionality, and Noise: Learnings From Field Trials
ABSTRACT
6.1. INTRODUCTION
6.2. DEPTH CALIBRATION
6.3. DIRECTIONALITY
6.4. NOISE
6.5. OVERCOMING THE FULL SUITE OF CHALLENGES – EXAMPLE FROM DEEP WATER
6.6. CONCLUSIONS
ACKNOWLEDGMENTS
REFERENCES
Note
7 Denoising Analysis and Processing Methods of Distributed Acoustic Sensing (DAS) Vertical Seismic Profiling (VSP) Data
ABSTRACT
7.1. INTRODUCTION
7.2. FIBER DEPLOYMENT TYPES AND NOISE SOURCES
7.3. CABLE RESONANCE REMOVAL
7.4. RANDOM NOISE SUPPRESSION
7.5. SNR ENHANCEMENT
7.6. CONCLUSION
REFERENCES
8 High‐Resolution Shallow Structure at Brady Hot Springs Using Ambient Noise Tomography (ANT) on a Trenched Distributed Acoustic Sensing (DAS) Array
ABSTRACT
8.1. INTRODUCTION
8.2. DATA AND METHODS
8.3. NCF RESULTS
8.4. DISPERSION MEASUREMENT RESULTS
8.5. SHEAR WAVE VELOCITY MODEL
8.6. CONCLUSIONS
ACKNOWLEDGMENTS
REFERENCES
9 Introduction to Interferometry of Fiber‐Optic Strain Measurements
ABSTRACT
9.1. INTRODUCTION
9.1.1. DAS Measurement Process
9.2. SENSITIVITY OF DAS TO FAR‐FIELD SOURCES
9.3 Sensitivity of DAS Cross‐Correlations to Plane Wave Sources
9.4. THOUGHT EXPERIMENT DEMONSTRATING AMBIENT NOISE INTERFEROMETRY TRENDS
9.4.1. Simple Case: Radial‐Radial Cross‐Correlations
9.4.2. Transverse‐Transverse Cross‐Correlations
9.5. SIMULATED AMBIENT NOISE INTERFEROMETRY ALONG CABLES
9.5.1. Signals Extracted Between two Parallel Fiber Cables
9.5.2. Virtual Source Perpendicular to Receiver Cable
9.6. CONCLUSIONS
ACKNOWLEDGMENTS
REFERENCES
10 Using Telecommunication Fiber Infrastructure for Earthquake Monitoring and Near‐Surface Characterization
ABSTRACT
10.1. INTRODUCTION
10.2. THE SFSO
10.3. CONTINUOUS MONITORING AND ANALYSIS OF LOCAL AND REGIONAL EARTHQUAKES
10.3.1. Weak Event Detection by Template Matching
10.3.2. Estimates of Event Amplitudes
10.4. CONTINUOUS MONITORING OF NEAR‐SURFACE CONDITIONS BY INTEFEROMETRY
10.4.1. Time‐Lapse Inteferometry Using Raleigh Waves Synthesized by Ambient Noise
10.4.2. Time‐Lapse Interferometry Using Surface Waves Generated by Quarry Blasts
10.5. IS THE COUPLING BETWEEN CABLES AND THE GROUND THE LIMITING FACTOR?
10.6. PROCESSING CHALLENGES FOR LARGE DAS ARRAYS IN URBAN ENVIRONMENTS
10.6.1. Analysis in Real Time of a Huge Stream of Data with Nonstationary and UnpredicTable Noise Sources
10.6.2. Automatic Identification and Muting of Bad Channels
10.6.3. Semiautomatic Determination of Virtual Receivers’ Location in DAS Arrays
10.7. CONCLUSIONS
ACKNOWLEDGMENTS
REFERENCES
11 Production Distributed Temperature Sensing versus Stimulation Distributed Acoustic Sensing for the Marcellus Shale
ABSTRACT
11.1. INTRODUCTION. 11.1.1. Marcellus Shale Energy and Environment Laboratory
11.1.2. Fiber‐Optic Technology and its Applications
11.2. METHODOLOGY
11.3. RESULTS AND DISCUSSIONS
11.4. CONCLUSIONS
ACKNOWLEDGMENTS
REFERENCES
12 Coalescence Microseismic Mapping for Distributed Acoustic Sensing (DAS) and Geophone Hybrid Array: A Model‐Based Feasibility Study
ABSTRACT
12.1. INTRODUCTION
12.2. DAS SYNTHETIC DATA FOR MICROSEISMIC EVENTS. 12.2.1. Expected Signature of DAS Microseismic Data
12.2.2. Simulation of DAS and Geophone Data for a Single Monitoring Array
12.3. THE LOCATION ALGORITHM FOR DAS-GEOPHONE HYBRID ARRAY
12.4. TESTS
12.4.1. Test Case 1: Monitoring Only at the Horizontal Section of the Array
12.4.2. Test Case 2: Monitoring by large aperture array
12.5. DISCUSSION AND CONCLUSION
REFERENCES
13 Continuous Downhole Seismic Monitoring Using Surface Orbital Vibrators and Distributed Acoustic Sensing at the CO2CRC Otway Project: Field Trial for Optimum Configuration
ABSTRACT
13.1. INTRODUCTION
13.2. PERMANENT MONITORING AT THE CO2CRC OTWAY PROJECT
13.3. FIELD EXPERIMENTS WITH DAS AND SOV SOURCES AT THE CO2CRC OTWAY PROJECT
13.4. OFFSET VSP PROCESSING
13.5. MAY 2017 FIELD TRIAL: CONVENTIONAL SINGLE‐MODE FIBER VS. CONSTELLATION FIBER
13.6. NOVEMBER 2017 FIELD TRIAL: PERFORMANCE OF SMALL AND LARGE MOTORS
13.7. SUMMARY AND CONCLUSIONS
ACKNOWLEDGMENTS
REFERENCES
14 Introduction to Distributed Acoustic Sensing (DAS) Applications for Characterization of Near‐Surface Processes
ABSTRACT
14.1. INTRODUCTION
14.2. CONSIDERATIONS FOR DEPLOYMENTS
14.2.1. A Continuous Sensor Array, Signal‐to‐noise Ratio, and Bandwidth
14.2.2. Signal‐to‐fiber Coupling
14.2.3. Economics for Near‐surface Characterization
14.3. SPECIFIC TOPICS IN THIS CHAPTER. 14.3.1. Ambient Noise Tomography
14.3.2. 4‐D Time‐lapse Imaging
14.3.3. Multichannel Analyses of Surface Waves
14.3.4. Utilization of Dark Fiber
14.3.5. Opportunities and Challenges
14.4. CONCLUSIONS
REFERENCES
15 Surface Wave Imaging Using Distributed Acoustic Sensing Deployed on Dark Fiber: Moving Beyond High‐Frequency Noise
ABSTRACT
15.1. INTRODUCTION
15.2. DARK FIBER NETWORKS: THE ESNET DARK FIBER TESTBED
15.3. STUDY SITE AND DATA ACQUISITION
15.4. DATA CHARACTERISTICS AND ANALYSIS OF NOISE SOURCES
15.5. PROCESSING STRATEGY
15.5.1. Data Selection
15.5.2. Ambient Noise Interferometry and Dispersion Analysis
15.5.3. Multimodal Inversion Using the Haskell‐Thomson Determinant Method
15.6. RESULTS. 15.6.1. Site Comparison Data
15.6.2. Inverted Vs Structure and Comparison with Ground Truth Data
15.7. DISCUSSION
15.8. CONCLUSIONS
ACKNOWLEDGMENTS
REFERENCES
16 Using Distributed Acoustic Sensing (DAS) for Multichannel Analysis of Surface Waves (MASW)
ABSTRACT
16.1. INTRODUCTION
16.2. DAS MEASUREMENT PRINCIPLES
16.3. STUDY AREA AND EQUIPMENT LAYOUT
16.4. LARGE SHAKER SEISMIC SOURCE
16.5. DAS AND GEOPHONE SENSORS
16.6. MULTICHANNEL ANALYSIS OF SURFACE WAVES (MASW)
16.7. SURFACE-WAVE DISPERSION ANALYSIS RESULTS
16.8. DISCUSSION
16.9. SUMMARY AND CONCLUSIONS
ACKNOWLEDGMENTS
REFERENCES
17 A Literature Review: Distributed Acoustic Sensing (DAS) Geophysical Applications Over the Past 20 Years
ABSTRACT
17.1. INTRODUCTION
17.2. FBG‐BASED QDAS GEOPHYSICAL APPLICATIONS
17.3. VARIOUS DAS GEOPHYSICAL APPLICATIONS. 17.3.1. DAS Principle, Instrument, Installation, Tests, and Advances
17.3.2. DAS Applications in VSP (Borehole Seismic)
17.3.3. DAS in Downhole Surveillance and Flow Monitoring
17.3.4. DAS in Monitoring Hydraulic Fracturing and Microseismicity
17.3.5. DAS in Carbon CCS and CO2 Injection Monitoring
17.3.6. DAS in Surface Seismic Exploration
17.3.7. DAS for Geothermal System, Mining, and Mineral Exploration
17.3.8. DAS Monitoring for Safety and Security
17.3.9. DAS for Near‐Surface and Earthquake Seismology, Fault Characterization, and Deformation
17.3.10. DAS Data Management, Processing, and Machine Learning
17.4. SOME THOUGHTS ON RECENT ADVANCES AND FUTURE APPLICATIONS
17.5. CONCLUSION
ACKNOWLEDGMENTS
REFERENCES
INDEX
WILEY END USER LICENSE AGREEMENT
Отрывок из книги
212 The Early Earth: Accretion and Differentiation James Badro and Michael Walter (Eds.)
.....
Directionality of the DAS response depends on the fiber optic cable configuration and the cable design, as the device itself is sensitive only to fiber elongation. We will start our consideration where the fiber is placed linearly inside a cable, with no slippage between fiber and cable, nor between the cable and the ground. In this case, fiber displacement will follow ground displacement, and sensitivity will depend on the relative position of fiber and seismic source. A similar mechanical principle was used for the electromagnetic linear strain seismograph to measure variations in the distance between two points of the ground (Benioff, 1935). DAS directional response with respect to incident angle Γ can be found by transformation of the strain tensor components with rotation using geometrical consideration. For a longitudinal (P) apparent wave, it will be cos2Γ, and for transversal (S) wave sin Γ cos Γ, similar to Benioff (1935) (see Figure 1.14). Detailed analysis and diagrams for Rayleigh and Love waves can be found in Martin et al. (2018).
In vertical seismic profiling (VSP), in the vertical part of the well, both cable and seismic waves are in the same direction for near‐offsets, so the DAS is more sensitive to P‐waves, in which the acoustic displacement vector coincides with the fiber direction. In other applications, such as fracking, the microseismic source is usually on a side of the cable, so shear waves can be effectively detected.
.....