Clathrate Hydrates
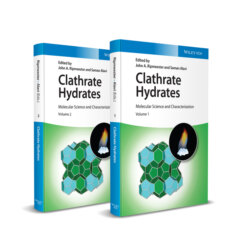
Реклама. ООО «ЛитРес», ИНН: 7719571260.
Оглавление
Группа авторов. Clathrate Hydrates
Table of Contents
List of Tables
List of Illustrations
Guide
Pages
Clathrate Hydrates. Molecular Science and Characterization
Clathrate Hydrates. Molecular Science and Characterization
Preface. Scope
1 An Introduction to Clathrate Hydrate Science
1.1 Introduction
1.2 Selected Highlights of Clathrate Hydrate Science Research Up to the Present
1.3 Clathrate Hydrate Research at the NRC Canada
1.4 Contributors to NRC Clathrate Hydrate Research
1.5 Review Articles and Books on Clathrate Hydrates
1.6 Conference Proceedings. 1.6.1 Canadian Permafrost Conference
1.6.2 Physics and Chemistry of Ice
1.6.3 International Conference on Gas Hydrates (IGCH) Proceedings
2 An Introduction to Clathrate Hydrates
2.1 Introduction
2.2 The First Gas Hydrates
2.3 The Phase Rule
2.4 de Forcrand and Villard – Career Gas Hydrate Researchers
2.5 Nikitin and von Stackelberg
2.6 Solving the Gas Hydrate Puzzle
2.7 Clathrate Hydrate Science – A New Era
2.8 Clathrate Hydrates in Engineering
2.9 Clathrate Hydrates in Nature
2.10 Summary and Observations
References
Notes
3 Classification of Clathrate Hydrates
3.1 Introduction
3.2 Hydrates as Clathrates
3.3 Clathrate and Related Hydrates – Guest Chemistry
3.4 The Canonical Clathrate Hydrates
3.4.1 Polyhedra and Filling Three‐Dimensional Space
3.4.2 Filling the Polyhedra
3.5 Phase Equilibria. 3.5.1 Simple Hydrates
3.5.2 Double and Mixed Hydrates, Natural Gas Hydrates
3.6 Tabulation of Hydrate Properties. 3.6.1 Simple Clathrate Hydrates
3.6.2 CS‐II (sII) Double Hydrates (Guests that Require a Help Gas for Stability)
3.6.3 HS‐III (sH) Hydrate Guests
3.7 Summary
References
4 Synthesis of Clathrate Hydrates
4.1 Introduction
4.2 General Considerations in the Synthesis of Clathrate Hydrates. 4.2.1 A Complex Process
4.2.2 Air Entrainment
4.3 Synthesis of Hydrates with Water‐Soluble Guests Near Ambient Conditions. 4.3.1 Hydrates with Congruent Melting Points
4.3.2 Hydrates with Incongruent Melting Points
4.4 Synthesis of Hydrates of Guests with Low Solubility in Water
4.4.1 Low‐Pressure Methods: Water–Liquid Guest and Water–Gaseous Guest Reactions
4.4.2 Powdered Ice Reactions with Liquid or Gaseous Guests
4.5 Synthesis of Clathrate Hydrates of Strongly Hydrated or Reactive Guests
4.6 Pure Hydrates – Kinetic and Thermodynamic Control
4.7 High‐Pressure Reactors. 4.7.1 Stirred Reactors
4.7.2 Stationary (Non‐stirred) Reactors
4.7.3 Other Setups for Hydrate Synthesis – Bubble Columns, Spray Reactors
4.8 Synthesis of Single Crystals
4.9 Summary
References
5 Structures of Canonical Clathrate Hydrates
5.1 Introduction
5.2 The Canonical Clathrate Hydrates. 5.2.1 General Structural Properties
5.2.2 Geometry of Unit Cells and Cages: CS‐I, CS‐II, and HS‐III
5.2.2.1 Structural Features CS‐I, CS‐II, and HS‐III Clathrate Hydrates
5.2.2.2 Correlation of Guest Size with Unit Cell Dimensions
5.2.2.3 Flexible Guest Molecules Showing Conformational Isomerism
5.2.2.4 Location of Guest Molecules in the Cages
5.2.2.5 Effects of Hydrogen Bonding on Cage Structure and Guest–Water Interactions
5.2.2.6 Halogen–Water Interactions in Clathrate Hydrates (Chlorine)
5.2.2.7 Polymorphism
5.2.3 Geometry of Unit Cell and Cages: Tetragonal Bromine Hydrate (TS‐I)
5.2.4 Geometry of Unit Cell and Cages: Dimethyl Ether Hydrate (TrS‐I)
5.2.5 Geometry of Unit Cell and Cages: Xe Hydrate (HS‐I)
5.3 Some General Structural Considerations
5.3.1 Tiling in Three‐Dimensional Space – Frank–Kasper and Weaire–Phelan Polyhedra
5.3.2 Schlegel Diagrams
5.3.3 Polytypism
5.3.3.1 Hydrate Structures as Layered Polytypes
5.3.4 Materials with Structural Features in Common with Clathrate Hydrates
References
6 Structures of Noncanonical Clathrates and Related Hydrates
6.1 Introduction
6.2 Amine Hydrates
6.3 Ionic Clathrate Hydrates. 6.3.1 Salt Hydrates
6.3.1.1 Salt Hydrates – Cations as Large Cage Guests
6.3.1.2 Salt Hydrates – Cations as Large Cage Guests, Neutral Small Cage Guests
6.3.1.3 Salt Hydrates – Cations as Small‐Cage Guests
6.3.2 Hydrates of Strong Acids
6.3.3 Hydrates of Strong Bases
6.3.4 Ionic Clathrate Hydrates with Heterogeneous Frameworks
6.3.5 Clathrates with H2O–NH4F Solid Solution Frameworks
References
7 Thermodynamics and Statistical Mechanics of Clathrate Hydrates
7.1 Introduction
7.2 Clathrate Hydration Numbers and Cage Occupancies
7.2.1 Direct Measurement of Hydration Numbers
7.2.2 Thermodynamic Methods to Determine Guest Occupancy. 7.2.2.1 The Clapeyron and Clausius–Clapeyron Equations and the Use of Phase Equilibria
7.2.2.2 The Miller–Strong Method and Effects of Solutes on Phase Equilibria
7.2.2.3 Calorimetry and Other Instrumental Methods in Conjunction with Thermodynamic Methods
7.3 Enthalpy of Dissociation of Hydrate Phases
7.4 Statistical Mechanics of Clathrate Hydrates: The van der Waals–Platteeuw Solid Solution Model for Clathrate Hydrate Formation
7.5 Application of the van der Waals–Platteeuw Theory to Determining Hydrate Equilibrium Composition
7.5.1 Using van der Waals–Platteeuw Theory to Determine Cage Occupancies
7.5.2 Instrumental Methods in Conjunction with the van der Waals–Platteeuw Theory to Determine Occupation Fractions
7.5.2.1 Solid‐State NMR
7.5.2.2 Raman Spectroscopy
7.5.2.3 Diffraction Methods
7.5.3 Some General Conclusions and Nonstoichiometry of Clathrate Hydrates
7.6 Computational Predictions of Hydrate Dissociation Pressures Using the van der Waals–Platteeuw Theory
7.7 Extensions of the van der Waals–Platteeuw Theory
7.7.1 Multiple Cage Occupancies and Guest Mixtures
7.7.2 Relaxing Some Position Restraints on Cage Water Molecules
7.7.3 Relaxing the Constraint of Constant Volume on the Hydrate Phase
7.7.4 Validity of the Basic van der Waals–Platteeuw Theory
7.8 Other Thermodynamic Topics. 7.8.1 Encagement Enthalpy
7.8.2 Thermodynamic Inhibitors to Hydrate Formation
7.8.3 Compositional Tuning in Clathrate Hydrates
7.8.4 Transitions Between Binary CS‐II and HS‐III Binary Hydrates to Pure CS‐I Hydrates for Small Guest Molecules
7.8.5 A Lower Critical Decomposition Temperature
7.9 Conclusions
References
8 Molecular Simulations of Clathrate Hydrates
8.1 Introduction
8.2 Molecular Simulations. 8.2.1 Classical Molecular Dynamics Simulations
8.2.2 Monte Carlo Simulations of Clathrate Hydrates
8.2.3 Ab Initio Molecular Dynamics Simulations
8.2.4 Classical Interaction Potentials for Simulating Clathrate Hydrates
8.2.5 Proton Arrangements in the Clathrate Hydrate Simulations
8.3 Structural Characterization of Clathrate Hydrates with Simulations
8.3.1 Radial Distribution Functions
8.3.2 Lattice Constants and Three‐Phase Equilibrium Lines
8.3.3 Guest Distribution and Structure in Cages
8.3.4 Order Parameters and Characterization of Clathrate Hydrate, Ice, and Water Phases
8.3.5 Guest–Host Hydrogen Bonding in Clathrate Hydrate Cages
8.4 Dynamic Characterizations of Guest Motion in Cages
8.4.1 Velocity and Orientation Autocorrelation Functions
8.5 Simulations of Clathrate Hydrates
8.5.1 Mechanisms of Hydrate Decomposition, Nucleation, and Growth
8.5.2 Enthalpy of Formation, Decomposition, and Encagement from Molecular Simulations
8.6 Ab Initio Quantum Mechanical Calculations of Clathrate Hydrates
8.6.1 Stationary Quantum States of Small Guests in Cages
8.6.2 Ab Initio Molecular Dynamics
8.7 Conclusions and Outlook
References
9 X‐ray and Neutron Diffraction and Scattering of Clathrate Hydrates
9.1 Introduction
9.2 Crystallography and X‐ray Diffraction
9.2.1 Comments on Diffraction as Applied to Hydrate Structure Determination
9.2.1.1 Single‐Crystal Diffraction
9.2.1.2 Powder Diffraction
9.3 Instrumentation
9.4 Structural Characterization with Diffraction Methods
9.4.1 Diffraction and Structure–Guest Size Relationship
9.4.2 Unconventional Applications of Diffraction
9.5 Neutron Diffraction or Elastic Neutron Scattering
9.6 Inelastic Neutron Scattering
9.7 Inelastic X‐ray Scattering
9.8 Summary
References
10 Characterization of Clathrate Hydrates Using Nuclear Magnetic Resonance Spectroscopy
10.1 Introduction
10.2 NMR Interactions
10.2.1 The Zeeman Interaction
10.2.2 Other Interactions
10.2.2.1 The Shielding Interaction (σ) and Chemical Shift (δ)
10.2.2.2 The Nuclear Dipole–Dipole Interaction
10.2.2.3 The Spin–Spin J‐Coupling Interaction
10.2.2.4 The Quadrupolar Coupling Interaction
10.2.2.5 The Spin–Rotation Coupling Interaction
10.2.2.6 Interactions with Unpaired Electrons
10.2.3 Units
10.3 Experimental Aspects of NMR Spectroscopy. 10.3.1 The Basic NMR Experiment
10.3.2 Techniques for Enhancing Sensitivity and Resolution. 10.3.2.1 Dipolar Decoupling
10.3.2.2 Magic Angle Spinning, MAS
10.3.2.3 Cross‐Polarization (CP)
10.3.2.4 Hyperpolarization of 129Xe (HP Xe)
10.4 The Development of NMR Techniques Over Time
10.5 NMR Powder Line Shapes in Clathrate Hydrates
10.5.1 Dipolar Line Shapes. 10.5.1.1 Magnetic Dilution
10.5.1.2 Two‐Spin Systems
10.5.1.3 Three‐Spin Systems
10.5.1.4 Four‐Spin Systems
10.5.1.5 Six‐Spin Systems
10.5.1.6 Multi‐Spin Systems
10.5.1.7 Effects of Paramagnetic Oxygen on 1H Line Shapes
10.5.2 Chemical Shift Line Shapes
10.5.2.1 129Xe NMR
10.5,2.1.1 Xenon in CS‐I
10.5.2.1.2 Xenon in CS‐II
10.5.2.1.3 Xenon in HS‐III
10.5.2.1.4 Xenon in TS‐I
10.5.2.1.5 Xenon in HS‐I
10.5.2.1.6 Xenon in TrS‐I
10.5.2.2 Chemical Shift Line Shapes of Other Nuclei: 77Se, 31P, 19F, 13C
10.5.3 Quadrupolar Line Shapes. 10.5.3.1 Spin 1: Deuterium 2H
10.5.3.2 Half‐Integer Quadrupolar Nuclei (131Xe, 83Kr, 33S, 17O)
10.5.3.2.1 17O NMR of the Water Molecules of the Hydrate Framework
10.5.3.2.2 131Xe NMR of Clathrate Hydrates
10.5.3.2.3 83Kr NMR of Clathrate Hydrates
10.5.3.2.4 33S NMR of Clathrate Hydrates
References
11 Specialized Methods of Nuclear Magnetic Resonance Spectroscopy and Magnetic Resonance Imaging Applied to Characterization of Clathrate Hydrates
11.1 Introduction
11.2 13C MAS NMR in Compositional and Structural Analysis of Gas Hydrates
11.2.1 Experimental Considerations
11.2.2 Overview of 13C MAS NMR in Clathrate Hydrates
11.2.3 Concluding Remarks and Outlook
11.3 129Xe NMR Applications: Other Topics. 11.3.1 Transient/Metastable Phases
11.3.2 Rapid Scanning of the Formation of CS‐I Xe Hydrate from Ice with Hyperpolarized Xe
11.3.3 Annealing of Co‐deposits of Xe and H2O
11.3.4 H2O‐NH4F Solid Solution Frameworks
11.4 Ionic Hydrates
11.4.1 Hydrates of Alkylammonium Salts
11.4.2 Hydrates of Strong Acids
11.4.3 Hydrates of Strong Bases
11.5 Clathrate Hydrates and Magnetic Resonance Imaging
11.5.1 Information About Gas Hydrates Accessible by Magnetic Resonance Imaging
11.5.2 Experimental Conditions and Equipment for MRI in Gas Hydrates
11.5.3 Overview of Current MRI Applications in Gas Hydrate Research
11.5.4 Concluding Remarks and Outlook
References
12 Reorientation and Diffusion in Clathrate Hydrates
12.1 Introduction
12.2 Early Work on Clathrates/Inclusion Compounds
12.3 Dynamics. 12.3.1 Dynamics and Timescales
12.3.2 Dielectric Relaxation
12.3.3 NMR Spectroscopy. 12.3.3.1 Nuclear Dipolar Coupling
12.3.3.1.1 Moment Analysis
12.3.3.1.2 NMR Relaxation Times
12.3.3.2 Nuclear Quadrupolar Interactions. 12.3.3.2.1 Line Shapes
12.3.3.2.2 Relaxation
12.3.3.3 Chemical Shift Line shapes
12.4 Water Dynamics in Ice and Clathrate Hydrates
12.4.1 Water Dynamics in Ice Ih
12.4.2 Water Dynamics in Clathrate Hydrates
12.5 Guest Motions. 12.5.1 Guest Reorientation: General Considerations
12.5.1.1 Reorientation of Spherical Top Guest Molecules
12.5.1.2 Reorientation of Symmetric Top Guest Molecules
12.5.1.3 Reorientation of Asymmetric Top Guest Molecules
12.5.1.3.1 Some Special Cases – Trimethylene Oxide (TMO), Ethylene Oxide (EO)
12.5.2 Diffusion
12.5.3 Nonclassical Dynamics
12.5.3.1 Methyl Groups
12.5.3.2 Dynamics of Light Tetrahedral Molecules
12.6 Summary
References
13 IR and Raman Spectroscopy of Clathrate Hydrates
13.1 Fundamentals and Quantification
13.2 IR Spectroscopy of Clathrate Hydrates. 13.2.1 Far IR Transmission – FT‐IR on Vapor‐Deposited Thin Films
13.2.2 Recent Studies of Clathrate Hydrates Using IR Spectroscopy
13.3 Raman Spectroscopy of Clathrate Hydrates
13.3.1 Guest Molecule Information
13.3.1.1 Detection of Encapsulation
13.3.1.2 Quantification of Raman Peak Positions
13.3.1.3 Quantification of Cage Occupancy
13.3.1.4 Application of Raman Spectroscopy to Kinetic Processes
13.3.1.5 Analysis of Natural Hydrate Samples
13.3.2 Noncontact, Non‐destructive Measurements of Gas Hydrates Via Visible Light
13.3.2.1 Application of Raman Spectroscopy to Clathrate Hydrate Kinetic Studies
13.3.2.2 Gas Hydrate Phases Obtained under High‐Pressure Conditions
13.3.2.3 In Situ Analysis of Natural Hydrate Sample Under Deep Sea Condition
13.4 Conclusions
Appendix 3A Raman Peaks of Clathrate Hydrate Guests
References
14 Kinetics of Clathrate Hydrate Processes
14.1 Introduction
14.2 Experimental Measurement of Hydrate Process Rates. 14.2.1 Kinetics – Gas Uptake Measurements
14.2.2 Kinetics of CS‐1 and CS‐II hydrates
14.2.3 Kinetics of HS‐III Hydrates
14.2.4 Kinetics Measurements – Other Methods
14.2.5 Average and Spatially Localized Kinetics
14.3 Modeling the Kinetics of Hydrate Nucleation
14.3.1 Hydrate Formation
14.3.2 Homogeneous Nucleation
14.3.3 Heterogeneous Nucleation
14.3.4 Validity and Relevance of Classical Nucleation Theory
14.4 Hydrate Phase Transformations
14.4.1 Hydrate Growth from Water
14.4.2 Hydrate Growth from Ice
14.4.2.1 The Shrinking Core Model
14.4.2.2 The Avrami Equation
14.4.3 Hydrate Crystal Morphology
14.4.4 Hydrate Decomposition
14.5 Metastability
14.6 Kinetic Modifiers
14.6.1 Surfactants
14.6.2 Defect Generation in the Hydrogen‐Bonded Ice and Hydrate Lattices
14.6.3 Kinetic Hydrate Inhibitors
14.6.3.1 Macroscopic Descriptions of Hydrate Inhibition
14.6.3.2 Mechanism of Kinetic Inhibition
14.6.3.3 Complexities of the Hydrate Inhibition Process
14.7 Molecular Simulations of Clathrate Hydrate Nucleation and Growth
14.7.1 Simulations of Heterogeneous Nucleation
14.7.2 Molecular Simulations of Homogeneous Nucleation
14.7.3 Simulations of Hydrate Growth
14.7.4 Simulations of Hydrate Growth and Decomposition in the Presence of Inhibitors
14.8 Concluding Remarks
References
15 Mechanical and Thermal Transport Properties of Clathrate Hydrates
15.1 Introduction
15.2 Theoretical Background. 15.2.1 Elastic Moduli
15.2.2 Thermal Conductivity
15.3 Mechanical Properties: Acoustic Velocity and Elastic Constants
15.4 Thermal Expansion
15.5 Transport Properties: Thermal Conductivity
15.6 Molecular Dynamics Simulations of Thermal Properties of Clathrate Hydrates
15.7 Summary
References
16 Applications of Clathrate (Gas) Hydrates
16.1 Introduction
16.2 Flow Assurance in Oil and Gas Pipelines
16.2.1 Large‐Scale Flow Loops
16.2.2 Catastrophic Hydrate Formation and Pipeline Plug Potential
16.2.3 Oil and Gas Pipelines with Hydrophobic Surfaces
16.3 Natural Gas Energy Recovery from the Earth's Hydrates
16.3.1 Extraction of Natural Gas by Injection of CO2 or CO2/N2 Flue Gas
16.4 Desalination
16.5 Concentration of Wastewater and Aqueous Organic Solutions
16.6 Storage and Transportation of Natural Gas, Hydrogen, and Other Materials
16.6.1 Natural Gas Storage
16.6.2 Hydrogen Storage
16.7 Gas Separations
16.7.1 Metrics
16.7.2 Separation of CO2 from Flue Gas Mixtures (Post‐Combustion Capture)
16.7.2.1 Impact of SO2
16.7.3 Separation of CO2 from Fuel Gas Mixtures (Pre‐Combustion Capture)
16.7.4 Other Gas Separations
16.8 Conclusions
References
Index
WILEY END USER LICENSE AGREEMENT
Отрывок из книги
Volume 1
Edited by
.....
The ability of methane hydrate to exist outside its usual thermodynamic range of stability was first reported in a calorimetric study of hydrate decomposition (Davidson et al. studies on the naturally occurring hydrate from Gulf of Mexico, 1986). Later this property became an important feature (self‐preservation or anomalous preservation) of the concept to use methane hydrate as a medium for the storage and transport of natural gas. Many laboratories contributed to the development of this concept, including contributions from NRC (Takeya 2001) on the guest dependence of the preservation process and the nature of the ice formed during the preservation process. A recent study showed that THF hydrate could be superheated by coating it with cyclopentane hydrate that has a higher melting point than THF hydrate.
As described earlier, a combination of powder diffraction and NMR spectroscopic results led to the characterization of HS‐III as a previously unknown clathrate hydrate family. Another Xe hydrate structure, previously known only hypothetically, was characterized in a similar way as HS‐III. The hydrate, known as HS‐I, is of similar composition as the Xe hydrates CS‐I and HS‐III and demonstrates that the synthetic pathway is important in defining the structure of the product.
.....