Handbook of Aggregation-Induced Emission, Volume 2
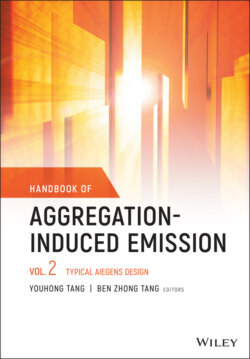
Реклама. ООО «ЛитРес», ИНН: 7719571260.
Оглавление
Группа авторов. Handbook of Aggregation-Induced Emission, Volume 2
Table of Contents
List of Tables
List of Illustrations
Guide
Pages
Handbook of Aggregation‐Induced Emission. Volume 2 Typical AIEgens Design
List of Contributors
Preface to Handbook of Aggregation‐Induced Emission
Preface to Volume 2: Typical AIEgens Design
1 Tetraphenylpyrazine‐based AIEgens: Synthesis and Applications
1.1 Introduction
1.2 Synthesis of TPP‐based AIEgens. 1.2.1 Cyclization Reaction
1.2.2 Suzuki–Miyaura Reaction
1.3 Functionalities of TPP‐based AIEgens
1.3.1 Organic Light‐emitting Diodes
1.3.2 Fluorescent Sensors
1.3.3 Chiral Cage for Self‐assembly to Achieve White‐light Emission
1.3.4 Metal–organic Framework
1.4 Conclusion
References
2 AIEgens Based on 9,10‐Distyrylanthracene (DSA): From Small Molecules to Macromolecules
2.1 Introduction
2.2 Application of AIE Luminogens Based on 9,10‐Distyrylanthracene. 2.2.1 Smart Materials with Stimulus Response
2.2.1.1 Piezofluorochromic Materials
2.2.1.2 Photochromic Materials
2.2.1.3 Thermochromic Materials
2.2.1.4 Acidichromic Materials
2.2.1.5 Multistimuli‐responsive Materials
2.2.2 High Solid‐state Luminescent Materials
2.2.3 Fluorescent Materials for Bioimaging
2.2.4 Fluorescent Probes for Chemical and Biological Sensing
2.2.4.1 Fluorescent Probes for Chemical Sensing
2.2.4.2 Fluorescent Probes for Biological Sensing
2.3 Conclusions and Outlook
Acknowledgments
References
3 Typical AIEgens Design: Salicylaldehyde Schiff Base
3.1 Introduction. 3.1.1 AIE and ESIPT of Salicylaldehyde Schiff Base
3.1.2 Universal Design of SSB‐based AIEgens
3.2 Fluorescent Probes. 3.2.1 Metal Ion Detection and Imaging
3.2.2 Biologically and Environmentally Related Molecular Detection and Imaging
3.2.3 Ratiometric pH Probes
3.2.4 Bioimaging
3.3 Fluorescent Materials. 3.3.1 Solid Fluorescence Emitting and Stimuli‐Responsive Materials
3.3.2 Nanoparticles
3.4 Summary and Perspectives
References
4 Diaminodicyanoquinodimethanes: Fluorescence Emission Enhancement in Aggregates and Solids
4.1 Introduction. 4.1.1 Molecular Materials
4.1.2 ‘Push–Pull’ Molecules
4.1.3 Diaminodicyanoquinodimethanes
4.2 Nonlinear Optical Materials based on DADQs. 4.2.1 Molecular Hyperpolarizability
4.2.2 SHG Materials
4.2.3 Structure–Property Correlations
4.3 Enhanced Fluorescence in Aggregates and Solids Based on DADQs
4.3.1 Remote Functionalized Systems
4.3.2 Color Tuning, Nanocrystals, and Colloids
4.3.3 Ultrathin Films
4.3.4 New Directions
4.4 Mechanistic Insights into the Enhanced Fluorescence. 4.4.1 Relevance of Intramolecular Effects
4.4.2 Role of Intermolecular Effects
4.5 Impact of Crystallinity on the Fluorescence Response
4.5.1 Amorphous‐to‐Crystalline Transformation: Fluorescence Switching and Tuning
4.5.2 Reversible Amorphous–Crystalline Transformations: Phase Change Materials
4.5.3 Impact of External Stimuli
4.6 Emergent and Potential Applications of DADQs. 4.6.1 Electroluminescence and Nonlinear Optics
4.6.2 Bioimaging
4.6.3 Photoelectrochemical and Photobioelectrochemical Applications
4.6.4 Memory Devices
4.7 Concluding Remarks
Acknowledgements
References
5 Aggregation‐induced Emission from the Sixth Main Group
5.1 Introduction
5.2 Oxygen
5.2.1 Oxygen‐Containing Heterocycles
5.2.2 Oxo‐ether Containing AIE‐Active Luminogens
5.3 Sulfur
5.3.1 Luminogens Based on Thiophenes
5.3.2 Thioethers with Aggregation‐Induced Emission Properties
5.3.3 Emissive Sulfones
5.4 Selenium and Tellurium
5.4.1 Selenium‐Containing Luminophores
5.4.2 Tellurium‐Containing Luminophores
5.5 Conclusion
Acknowledgment
References
6 Fluorescence Detection of Dynamic Aggregation Processes Using AIEgens: Hexaphenylsilole and Cyanostilbene
6.1 Introduction
6.2 Selective Detection of Phase Transformation During Evaporative Crystallization of Hexaphenylsilole
6.3 Observation of the Initial Stage of Organic Crystal Formation During Solvent Evaporation Using a Cyanostilbene Derivative
6.4 Chemometrix Analysis of the Aggregated Structure of Cyanostilbene in a Reprecipitation Solution Using Fluorescence Excitation Spectroscopy
6.5 UV‐triggered Fluorescence Enhancement of a Dicyanostilbene Derivative Film Cast from an Ethanol Solution
6.6 Concluding Remarks
Acknowledgments
References
7 Cyclic Triimidazole Derivatives: An Intriguing Family of Multifaceted Emitters
7.1 Introduction
7.2 The Protoype: Cyclic Triimidazole
7.3 Halogenated Derivatives of Cyclic Triimidazole
7.3.1 Bromine Derivatives
7.3.2 Iodine Derivatives
7.4 Organic Derivatives
7.4.1 2‐Fluoropyridine Derivative
7.4.2 Tribenzoimidazole Derivative
7.5 Hybrid Inorganic/Organic Derivatives
7.6 Conclusions
Acknowledgments
References
8 Synthesis of Multi‐phenyl‐substituted Pyrrole (MPP)‐based AIE Materials and Their Applications
8.1 Introduction
8.2 Modular Approach: Systematic Synthesis of MPPs
8.3 Structures and Photophysical Properties
8.4 Applications of MPP‐based Materials. 8.4.1 Chemical/Biological Sensing
8.4.2 Multi‐stimulus Response Materials
8.4.3 Optoelectronic Systems
8.4.4 Biological Application
8.5 Conclusion and Outlook
References
9 Development of a New Class of AIEgens: Tetraarylpyrrolo [3,2‐b] Pyrroles (TAPPs)
9.1 Introduction
9.2 The Accidental Discovery of TAPP
9.3 Synthesis of TAPP
9.4 Possible Mechanism of TAPP Synthesis
9.5 Reactivity of TAPP
9.6 π‐Expansion of TAPP
9.7 π‐Expanded 1,4‐dihydropyrrolo[3,2‐b]pyrrole
9.8 Photophysical Optical Properties of TAPP
9.9 Conclusion and Outlook
Acknowledgments
References
10 Small Molecule Organogels from AIE Active α‐Cyanostilbenes
10.1 Introduction
10.2 Organogels with Trifluoromethyl Substitution
10.3 Organogels with Chiral Units/Chiral Hosts
10.4 Stimuli–Responsive Organogels
10.5 Organogels with Sensing Applications
10.6 Concluding Remarks
Acknowledgments
References
11 Stimuli‐responsive Pure Organic Luminescent Supramolecules
11.1 Introduction
11.2 Pure Organic Fluorescent Supramolecules. 11.2.1 Pure Organic Fluorescent Supramolecules Containing Macrocycles
11.2.1.1 Pure Organic Fluorescent Supramolecules Containing Cyclodextrins
11.2.1.2 Pure Organic Fluorescent Supramolecules Containing Calixarenes
11.2.1.3 Pure Organic Fluorescent Supramolecules Containing Cucurbiturils
11.2.1.4 Pure Organic Fluorescent Supramolecules Containing Pillararene
11.2.1.5 Pure Organic Fluorescent Supramolecules Containing Crown Ether
11.2.2 Pure Organic Fluorescent Supramolecules Without Macrocycles
11.3 Pure Organic Phosphorescent Supramolecules. 11.3.1 Pure Organic Phosphorescent Supramolecules Based on Macrocyclic Molecules
11.3.1.1 Pure Organic Phosphorescent Supramolecules Containing Cyclodextrin
11.3.1.2 Pure Organic Phosphorescent Supramolecules Containing Cucurbiturils
11.3.1.3 Pure Organic Phosphorescent Supramolecules Containing Calixarenes
11.3.1.4 Pure Organic Phosphorescent Supramolecules Containing Crown Ether
11.3.2 Pure Organic Phosphorescent Supramolecules Without Macrocyclic Molecules. 11.3.2.1 Pure Organic Supramolecular Phosphorescence System With Doping‐Based Host–Guest Interaction
11.3.2.2 Other Pure Organic Phosphorescent Supramolecules
11.4 Conclusions
Acknowledgments
References
12 AIE Fluorescent Polymersomes
12.1 Introduction
12.2 Structural Consideration of Block Copolymers for Polymersome Formation
12.3 Methods of Polymersome Preparation
12.4 Techniques of Polymersome Characterization
12.5 AIE Polymersomes Based on PEG‐b‐POSS
12.6 AIE Polymersomes Based on Amphiphilic Polypeptoids
12.7 AIE Polymersomes Based on PEG‐b‐Polycarbonate
12.8 AIE Polymersomes Based on Amphiphilic Polynorbornene
12.9 AIE Polymersomes Based on Amphiphilic Block Copolymers by RAFT Polymerization
12.10 Summary and Perspectives
References
13 Designs for AIE Molecules and Functional Luminescent Materials Based on Boron‐containing Element‐blocks
13.1 Introduction. 13.1.1 Generals of Commodity Luminescent Boron Complexes
13.1.2 Trends in the Development of Advanced Organic Electronic Devices
13.1.3 Strategies for Obtaining Solid‐state Luminescence and Stimuli‐responsiveness
13.1.4 New Ideas for Material Design Based on “Element‐blocks”
13.2 Solid‐state Luminescence and Luminochromism of o‐Carboranes. 13.2.1 Emission Mechanism of Aryl‐modified o‐Carboranes
13.2.2 AIE Behavior of o‐Carborane Materials
13.2.3 Formation of Twisted Intramolecular Charge Transfer (TICT) State in the Crystalline State of o‐Carboranes
13.2.4 Thermochromic Luminescence of o‐Carboranes
13.2.5 Intense Solid‐state Luminescent Molecules
13.2.6 Solid‐state Excimer Emission
13.3 Boron Complexes with β‐Ketimine and β‐Diketimine Ligands. 13.3.1 Generals of Boron Ketiminates and Diketiminates
13.3.2 Unique Solid‐state Luminescent Properties of Conjugated Boron Complexes
13.3.3 Thermally Stable Mechanochromic Luminescent Hybrid with the Siloxane Unit
13.3.4 Luminescent Properties of β‐Diketiminate Complexes
13.3.5 AIE‐active Conjugated Polymers
13.3.6 Design for Film‐type Sensors
13.3.7 Sensitive Luminochromic Sensors with Gallium Complexes
13.4 Rational Design for AIE‐active Molecules Based on “Flexible” Boron Complexes. 13.4.1 Concept for Rational Design
13.4.2 Ring‐fused or Nonring‐fused Molecules
13.4.3 Thermosalient‐active Molecules
13.4.4 Solid‐state Luminescent π‐Conjugated Polymer
13.5 Conclusion
References
14 Aggregation‐induced Emission (AIE) Active Metal–Organic Coordination Complexes
14.1 Introduction
14.2 Conception and Design Strategy
14.3 AIE Active Metallacycles
14.3.1 AIE Active Simple Metallacycles
14.3.2 AIE Active Fused Metallacycles
14.3.3 AIE Active Metallacycle Polymers
14.4 AIE Active Metallacages
14.5 AIE Active Metal–organic Frameworks (MOFs)
14.6 Summary and Outlook
Acknowledgments
References
15 AIE‐type Luminescent Metal Nanoclusters
15.1 Introduction
15.2 In the “Single‐cluster” Scenario. 15.2.1 AIE‐type Luminescent Metal NCs
15.2.2 Atomically Precise AIE‐type Luminescent Metal NCs
15.2.3 Approaches to Luminescence Enhancement of Metal NCs in the Scheme of AIE
15.2.3.1 Surface Engineering. 15.2.3.1.1 Ligands Rigidifying
15.2.3.1.2 Ligands Optimizing
15.2.3.2 Roles of the Core
15.3 Beyond the “Single‐cluster” Scenario
15.3.1 Poor‐solvent‐induced AIE of Metal NCs
15.3.2 Ion‐induced AIE of Metal NCs
15.3.3 Supramolecular Interactions Induced AIE of Metal NCs
15.3.4 Spatial Confinement‐induced AIE of Metal NCs
15.4 Application of the AIE‐type Luminescent Metal NCs
15.4.1 Chemical Sensing
15.4.2 Biological Applications
15.4.3 Photosensitizer
15.4.4 Light‐emitting Diodes (LEDs)
15.5 Conclusion and Outlook
References
16 Aggregation‐induced Emission in Coinage Metal Clusters
16.1 Introduction
16.2 AIE‐active Gold Cluster
16.3 AIE‐active Silver Cluster
16.4 AIE‐active Copper Cluster
16.5 AIE‐active Bimetallic Cluster
16.6 Conclusions
References
17 Activated Alkynes in Metal‐free Bioconjugation
17.1 Introduction
17.2 Alkyne–Azide‐based Bioconjugation
17.3 Activated Alkyne–Amine‐based Bioconjugation
17.4 Activated Alkyne–Thiol‐based Bioconjugation
17.5 Activated Alkyne–Hydroxyl‐based Bioconjugation
17.6 Activated Alkyne‐based Bioconjugation and Polymerization in Living Cells and Pathogens
17.7 Conclusion
References
18 AIE‐active BODIPY Derivatives
18.1 Introduction
18.2 Structures of BODIPY Derivatives
18.2.1 BODIPY Derivatives Without Other Chromophore
18.2.2 TPE‐containing BODIPYs
18.2.3 TPA‐containing BODIPYs
18.2.4 Benzodithiophene‐containing BODIPYs
18.2.5 Chiral BODIPYs
18.2.6 Metal‐containing BODIPYs
18.2.7 BODIPY‐containing Polymers
18.2.8 Other BODIPY Derivatives
18.3 Structural–property Relationship
18.3.1 Conjugation Effect
18.3.2 Number and Position of Substitutes
18.3.3 Substitution Group
18.3.4 Alkyl Substitutes on BODIPY Core
18.3.5 AIEgens Attached Through Nonconjugated Spacers
18.3.6 Other Substitution Structures
18.4 Application
18.4.1 Chemosensor
18.4.2 Bioimaging
18.5 Conclusion
References
19 Photochemistry‐regulated AIEgens and Their Applications
19.1 Introduction
19.2 Photocleavage Reaction
19.3 Photoreduction Reaction
19.4 Photocyclodehydrogenation Reaction
19.5 Photooxidative Dehydrogenation Reaction
19.6 Spiropyran‐merocyanine Reversible Conversion
19.7 Dithienylethene‐based Ring‐open/‐closing Reaction
19.8 Enol–Keto Isomerization Reaction
19.9 E/Z Isomerization Reaction
19.10 Photo‐induced [2 + 2] Cycloaddition
19.11 Combinational Photoreactions
19.12 Conclusion and Outlook
References
20 Design and Development of Naphthalimide Luminogens
20.1 Introduction
20.2 Naphthalimides with N‐Functionalization (I)
20.3 Naphthalimides Substituted at the 4th Position with Oxygen Atom (II)
20.4 Naphthalimides Substituted at the 4th Position with Nitrogen Atom (III)
20.5 Naphthalimides with C−C Aromatic Substitution (IV)
20.6 Naphthalimides with C−C Double‐ and Triple‐Bond Substitutions (V and VI)
20.7 Naphthalimides with the Significant Role of Multifunctionalization (VII)
20.8 Conclusion and Outlooks
References
Index. a
b
c
d
e
f
g
h
i
j
l
m
n
o
p
q
r
s
t
u
v
w
x
WILEY END USER LICENSE AGREEMENT
Отрывок из книги
Edited by
Youhong Tang
.....
A polysaccharide SSB sensor for facile, sensitive, and selective heparin detection has also been fabricated [48]. Heparin is a mucopolysaccharide composed of D‐β‐glucuronic acid and N‐acetylglucosamine to form a repeating disaccharide unit. Its skeleton has many anionic groups (such as carboxyl and sulfonic acid groups, etc.), making heparin highly negatively charged. As a medicinal anti‐hemagglutinating agent as well as a special antidote, heparin is hence of great significance for analytical detection. As shown in Figure 3.20a, salicylazine 33 is modified with two positively charged tertiary amine groups, which can be combined with negatively charged heparin through a charge–charge interaction. The emission of probe 33 in the Tris‐HCl buffer solution at pH 7.0 was extremely weak may be and its emission at 530 nm increased rapidly upon the addition of heparin (Figure 3.20b), which was due to the aggregation through electrostatic interactions. When the concentration of heparin reached 22 μg/ml, a fluorescence enhancement of about 40‐folds had been detected. The linear range is 0.2–14 μg/ml, the detection limit is 57.6 ng/ml, and the response time is as short as 2 minutes. Figure 3.20c also represents good selectivity of probe 33 for heparin from other polysaccharides such as chondroitin sulfate (ChS), hyaluronic acid (HA), and dextran (DeX).
Figure 3.20 (a) Design principle of the fluorescence turn‐on detection of heparin based on AIE characteristics of 33. (b) Fluorescence spectra of 33 in the presence of different amounts of heparin (from 0 to 22 μg/ml), λex = 391 nm. (c) The fluorescence intensity of 33 in the presence of different amounts of HA, DeX, ChS, and heparin.
.....