Phosphors for Radiation Detectors
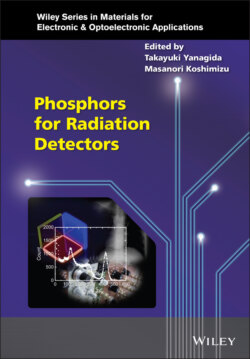
Реклама. ООО «ЛитРес», ИНН: 7719571260.
Оглавление
Группа авторов. Phosphors for Radiation Detectors
Table of Contents
List of Tables
List of Illustrations
Guide
Pages
Wiley Series in Materials for Electronic and Optoelectronic Applications
Phosphors for Radiation Detectors
List of Contributors
Preface
Series Preface. Wiley Series in Materials for Electronic and Optoelectronic Applications
1 Ionizing Radiation Induced Luminescence
1.1 Introduction
1.2 Interactions of Ionizing Radiation with Matter
1.3 Scintillation. 1.3.1 Energy Conversion Mechanism
1.3.2 Emission Mechanism
1.3.3 Scintillation Light Yield and Energy Resolution
1.3.4 Timing Properties
1.3.5 Radiation Hardness
1.3.6 Temperature Dependence
1.4 Ionizing Radiation Induced Storage Luminescence. 1.4.1 General Description
1.4.2 Analytical Description of TSL
1.4.3 Analytical Description of OSL
1.5 Relationship of Scintillation and Storage Luminescence
1.6 Common Characterization Techniques of Ionizing Radiation Induced Luminescence Properties
References
2 Organic Scintillators
2.1 Introduction
2.2 Basic Electronic Processes in Organic Scintillators
2.2.1 Electronic States and Excited States Dynamics of Organic Molecules
2.2.2 Excitation Energy Transfer
2.2.3 Scintillation Dynamics in Organic Scintillators at High Linear Energy Transfer
2.3 Liquid Scintillators
2.4 Organic Crystalline Scintillators
2.5 Plastic Scintillators
2.6 Organic–Inorganic Hybrid Scintillators
2.6.1 Loaded Organic Scintillators
2.6.2 Organic–Inorganic Nanocomposite Scintillators
References
3 Inorganic Oxide Scintillators
3.1 Introduction
3.2 Crystal Growth
3.3 Outlines of Oxide Scintillators
3.4 Silicate Materials. 3.4.1 Ce:Gd2SiO5 (Ce:GSO)
3.4.2 Ce:Lu2SiO5 (Ce:LSO)
3.4.3 Ce:Gd2Si2O7 (Ce:GPS)
3.4.4 LPS
3.5 Garnet Materials. 3.5.1 Ce:Y3Al5O12 (Ce:YAG)
3.5.2 Ce:Lu3Al5O12 (Ce:LuAG), Pr:Lu3Al5O12 (Pr:LuAG)
3.5.3 Ce:Gd3Al2Ga3O12 (Ce:GAGG)
3.5.4 Ce:Tb3Al5O12 (Ce:TAG)
3.6 Perovskite Materials. 3.6.1 Ce:YAlO3 (Ce:YAP)
3.6.2 Ce:LuAlO3 (Ce:LuAP)
3.7 Materials with Intrinsic Luminescence. 3.7.1 CdWO4
3.7.2 Bi4Ge3O12 (BGO)
3.7.3 PbWO4
References
4 Inorganic Fluoride Scintillators
4.1 Introduction
4.2 Crystal Growth of Fluorides. 4.2.1 Classification of Methods for Crystal Growth
4.2.2 Furnace Materials, Atmosphere, and Scavengers for Fluoride Crystal Growth
4.2.3 Fluoride Crystal Growth Methods by Pulling Out from the Melt
4.2.4 Fluoride Crystal Growth Methods by Solidifying the Melt in the Crucible
4.2.5 Fluoride Crystal Growth Methods Without Using Crucibles
4.3 Outline of Fluoride Scintillators
4.4 Fluoride Scintillators for γ‐Ray Detection. 4.4.1 Fluoride Scintillators Based on Luminescence from 5d‐4f Transitions of Ce3+ Ions
4.4.2 Fluoride Scintillators Based on Core‐Valence Luminescence
4.4.3 VUV Emitting Fluoride Scintillators Doped with Nd3+, Er3+, and Tm3+ Ions
4.5 Fluoride Scintillators for Neutron Detection. 4.5.1 Review for Neutron Scintillators
4.5.2 LiCaAlF6 Single Crystals
4.5.3 LiF/CaF2 Eutectic Composites
4.6 Fluoride Scintillators for Charged Particle Detection. 4.6.1 Methods for Charged Particle Detection
4.6.2 CaF2 Based Scintillators for Charged Particle Detection
References
5 Inorganic Halide Scintillators
5.1 Introduction: History of Inorganic Halide Scintillator Research and Development
5.2 Characteristics of Halide Materials. 5.2.1 Formation of Color Center and Self‐Trapped Exciton
5.2.2 Hygroscopicity
5.3 Basic Techniques for Halide Scintillation Crystal Growth
5.4 Novel Ternary and Quaternary Halide Scintillators. 5.4.1 Alkali Halide‐Rare Earth Halide (AX–REX3)
5.4.2 Alkali Halide‐Alkalin Earth Halide (AX–AEX2)
5.4.3 Elpasolite
5.5 Mixed‐Anion Halide Scintillators
5.6 Next Generation of Halide Scintillators. 5.6.1 Hf‐ and Tl‐Based Halide Scintillators
References
6 Semiconductor Scintillators
6.1 Introduction
6.2 Photoluminescence and Scintillation Mechanisms in Semiconductors
6.3 Various Semiconductor Scintillators
6.3.1 Undoped Semiconductor Scintillator
6.3.2 Doped Semiconductor Scintillator
6.3.2.1 Semiconductor Scintillator with Isoelectronic Impurities
6.3.2.2 Semiconductor Scintillators with Donor Impurities
6.3.2.3 Semiconductor Scintillators with Acceptor Impurities
6.3.2.4 Semiconductor Scintillators with a Donor–Acceptor Pair
6.4 Quantum Size Effect
6.5 Organic–Inorganic Perovskite‐Type Compounds. 6.5.1 Introduction
6.5.2 Materials and Structures
6.5.3 Sample Preparation
6.5.4 Fundamental Optical Property
6.5.5 Scintillation
6.5.5.1 Scintillation Light Yield
6.5.5.2 Scintillation Decay Time
6.5.5.3 Afterglow
References
7 Thermally Stimulated Luminescent (TSL) Materials
7.1 Introduction
7.2 TSL Phenomenon. 7.2.1 Basic Principles of TSL
7.2.2 Theory and Measurement of Glow Curves. 7.2.2.1 Theory of Glow Curves. 7.2.2.1.1 First‐Order Kinetics
7.2.2.1.2 Second‐Order Kinetics and General‐Order Kinetics
7.2.2.1.3 Relationship Between Glow Curve and Parameters
7.2.2.2 Measurement of Glow Curves
7.3 TSL Materials: Fluoride, Oxides, Sulfates, and Borate. 7.3.1 Fluorides. 7.3.1.1 Lithium Fluoride (LiF)
7.3.1.2 Calcium Fluoride (CaF)
7.3.2 Oxides. 7.3.2.1 Aluminum Oxide (Al2O3)
7.3.2.2 Beryllium Oxide (BeO)
7.3.3 Sulfates. 7.3.3.1 Calcium Sulfate (CaSO4)
7.3.4 Borates
7.3.4.1 Lithium Borate (Li2B4O7)
7.3.4.2 Magnesium Borate (MgB4O7)
7.4 TSL Dosimetric Properties for Photons, Charged Particles, and Neutrons
7.4.1 TSL Dosimetric Properties for Photons
7.4.2 TSL Dosimetric Properties for Charged Particles
7.4.3 TSL Dosimetric Properties for Neutrons
7.5 Two‐Dimensional (2‐D) TSL Dosimetry. 7.5.1 Introduction
7.5.2 Types of 2‐D TSLDs
7.5.3 Measurement Systems
7.5.4 Application of 2‐D TSLDs in Photon Beam Radiotherapy. 7.5.4.1 TSL Foils
7.5.4.2 TSL Slab
7.5.4.3 Ceramic TSL Slab
7.5.5 Outlook for 2‐D TSLDs
References
8 Optically‐Stimulated Luminescent Dosimeters
8.1 Introduction
8.2 Principles of OSL Phenomenon
8.3 OSL Materials and Dosimeters
8.4 Applications of OSL
8.5 Future Perspective
References
9 Radiophotoluminescence (RPL)
9.1 Introduction
9.2 RPL Phenomenon and the Definition
9.3 RPL Materials and Applications. 9.3.1 Introduction
9.3.2 Ag‐Doped Sodium‐Aluminophosphate Glasses. 9.3.2.1 Introduction
9.3.2.2 Principal Properties
9.3.2.3 Application in Personnel and Environmental Dose Monitoring
9.3.2.4 Other Applications in R&D
9.3.3 Al2O3:C,Mg. 9.3.3.1 Introduction
9.3.3.2 Principal Properties
9.3.3.3 Fluorescent Nuclear Track Detector
9.3.4 LiF. 9.3.4.1 Introduction
9.3.4.2 Principal Properties
9.3.4.3 Disk‐Type Imaging Plate
9.3.4.4 Fluorescent Nuclear Track Detector
9.3.5 Sm‐Doped Compounds. 9.3.5.1 Introduction
9.3.5.2 Principal Properties
9.3.5.3 Application in Microbeam Radiation Therapy (MRT)
9.3.6 Other RPL Materials
9.4 Conclusions
References
10 New Materials for Radiation Detectors: Transparent Ceramics
10.1 Introduction of Transparent Ceramic Materials. 10.1.1 Light Scattering Sources in Ceramics
10.1.2 History and Applications on Transparent Ceramics
10.2 Preparation Methodology. 10.2.1 Sintering Mechanism of Ceramics
10.2.1.1 Initial Stage
10.2.1.2 Intermediate Stage
10.2.1.3 Final Stage
10.2.2 Effect of Residual Pores
10.2.3 Preparation Methods of Transparent Ceramics
10.3 Transparent Materials
10.4 Transparent Ceramic Scintillator
10.4.1 Sesquioxide (Such as Y2O3, Gd2O3, and Lu2O3)
10.4.2 Gd2O2S (GOS)
10.4.3 Garnet Materials (Such as YAG, LuAG, and GAGG)
10.4.4 Lu2SiO5 (LSO)
10.4.5 SrHfO3
10.4.6 La2Zr2O7 and La2Hf2O7
10.4.7 ZnO
10.4.8 BaF2
10.4.9 CeF3
10.4.10 CsBr
10.4.11 LaBr3
10.4.12 SrI2
10.5 Transparent Ceramics for Dosimeter
10.5.1 Al2O3
10.5.2 CaF2
10.5.3 MgO
10.5.4 MgF2
10.5.5 CsBr
10.5.6 Y3Al5‐xGaxO12 (YAGG)
References
11 Luminescence in Glass‐Based Materials by Ionizing Radiation
11.1 Introduction
11.2 Structural and Physical Properties of Glass
11.3 Attenuation of Quantum Beam as Shielding Materials
11.4 Defect Formation in Oxide Glass by Quantum Beam Irradiation
11.5 Scintillation in Oxide Glass
11.5.1 Glass Scintillators for X‐Ray and γ‐Ray
11.5.2 Glass Scintillators for Neutrons
11.5.3 Storage Luminescence in Glass
11.6 Scintillation and Dosimetry in Non‐oxide Glass
11.7 Preparation of Glass
11.7.1 Melt Process
11.7.2 Vapor Process and Fiber Drawing
11.7.3 Liquid Process
11.8 Future Prospectives for Glass‐Based Materials
Acknowledgement
References
12 Detectors Using Radiation Induced Luminescence
12.1 Introduction
12.2 General Issues to Manufacturing the Detector
12.3 Scintillation Detectors for Gamma‐Rays and X‐Rays
12.3.1 Gamma‐Ray Spectrometer
12.3.2 Survey Meter and Area Monitor
12.3.3 Scintillation Detectors for Medical Applications
12.3.3.1 X‐Ray Flat Panel Detectors (FPDs)
12.3.3.2 X‐Ray Tomography (CT)
12.3.3.3 Single Photon Emission Computed Tomography (SPECT)
12.3.3.4 Emission Tomography (PET)
12.3.4 Scintillation Detectors for Other Applications
12.3.4.1 Nuclear Well Logging
12.3.4.2 Security
12.4 Scintillation Detectors for Charged Particles
12.5 Scintillation Detectors for Neutrons
12.5.1 Thermal Neutron Detectors
12.5.1.1 Medical Applications: Boron Neutron Capture Therapy
12.5.1.2 Security Applications: Radiation Portal Monitor
12.5.1.3 Material Science Applications: Neutron Imaging
12.5.2 Fast Neutron Detectors
12.6 Personal Dosimeters
12.6.1 TL‐Based Dosimetry System
12.6.2 OSL‐Based Dosimetry System
12.6.3 RPL‐Based Dosimetry System
12.7 OSL‐Based Imaging System
References
Index
WILEY END USER LICENSE AGREEMENT
Отрывок из книги
www.wiley.com/go/meoa
.....
(1.31)
respectively. In these equations, dn1/dt and dm1/dt represent a charging (trapping) process, and (η + ζ)nm and Βm nm1 represent dissipation processes. Let us consider the energy dissipation after stopping the irradiation at temperature T. In this case, if we assume J = 0 in (1.27)–(1.30) at time t = 0, then TL from the electron centers by electron release is
.....