Perovskite Materials for Energy and Environmental Applications
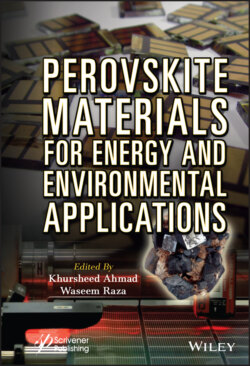
Реклама. ООО «ЛитРес», ИНН: 7719571260.
Оглавление
Группа авторов. Perovskite Materials for Energy and Environmental Applications
Table of Contents
List of Illustrations
List of Tables
Guide
Pages
Perovskite Materials for Energy and Environmental Applications
Preface
1. Computational Approach for Synthesis of Perovskite Solar Cells
1.1 Introduction
1.2 Preliminary Steps (I) Physical Basis for Semiconductor Device Modeling. A. Semiconductor at Thermal Equilibrium
Carrier Concentration
Intrinsic carrier concentration
Donors and acceptors
B. Nonequilibrium carrier concentration
(II) Basic equations for semiconductor modeling
Poisson’s Equation
Continuity equations
Current density equations
Optical generation of electron-hole pairs
(III) Recombination phenomenon in semiconductors
A. Radiative recombination
B. Auger recombination
C. Shockley-Read-Hall recombination (SRH)
1.3 Advanced Semiconductor Analysis (ASA)
1.4 Analysis of Microelectronic and Photonic Structures (AMPS)
1.5 Automat for Simulation of Heterostructures (AFORS-HET)
1.6 Solar Cell Capacitance Simulator (SCAPS)
1.7 Conclusion
References
2. Fundamentals of Perovskite Solar Cells
2.1 Introduction
2.2 Structure
2.3 Working Mechanism of PSC
2.4 Device Architecture
2.4.1 Mesoporous Structure
2.4.2 Planar Heterostructures
2.5 Properties. 2.5.1 High Optical Absorption
2.5.2 High Open-Circuit Voltage
2.5.3 Low Recombinations
2.5.4 Tunable Bandgap
2.5.4.1 Organic Cation (A)
2.5.4.2 Metal Cation (M)
2.5.4.3 Halide Anion (X)
2.5.5 Rapidly Increasing Efficiency
2.6 Drawbacks and Ongoing Challenges of PSCs. 1. Pb-free alternatives/toxicity of Pb
2. Stability
2.7 Conclusion
Acknowledgment
References
3. Surface Morphological Effects on the Performance of Perovskite Solar Cells
3.1 Introduction
3.2 Morphology Control
3.2.1 The Effect of Device Architecture on the Morphology and the Device Performance
3.2.2 Effect of Deposition Technique on the Morphology of the Perovskite Layer
3.2.2.1 One-Step Deposition Method
3.2.2.2 Two-Step Deposition Technique
3.2.2.3 Dual-Source Precursor Approach
3.2.2.4 Vacuum Deposition Technique
3.3 Effect of Various Parameters on Growth of Perovskite. 3.3.1 Effect of Solvent Additive
3.3.2 Effect of Solid Additive
3.3.3 Seed-Induced Growth of Perovskites
3.3.4 Homogenous Cap-Induced Crystallization
3.3.5 Effect of Hydrophobicity
Growth mechanism of perovskite films on hydrophilic vs hydrophobic surfaces:
3.3.6 Effect of Interface Modification
3.3.7 Effect of Solvent Annealing
References
4. Advanced Synthesis Strategies for Single Crystal Perovskite Halides
4.1 Introduction
4.2 Popular Single Crystal Growth Techniques
4.2.1 Anti-Solvent Vapor-Assisted Crystallization (AVC) Method
4.2.2 Inverse Temperature Crystallization (ITC)
4.2.3 Modified Inverse Temperature Crystallization
4.2.4 Solution Temperature Lowering Method
4.2.4.1 Top-Seeded Solution Growth Method
4.2.4.2 Bottom-Seeded Solution Growth Method
4.2.5 Bridgman (BG) Method
4.3 Other Techniques
Conclusions
References
5. Synchrotron-Based Techniques for Analysis of Perovskite Solar Cells
5.1 Introduction
5.2 Synchrotron Techniques, Their Limitations and Advantages
5.3 Synchrotron Radiation X-Ray Diffraction/Scattering (SR-XRD)
5.4 In Situ XRD
5.5 Small-Angle X-Ray Scattering
5.6 Wide-Angle X-Ray Scattering
5.7 Synchrotron Radiation-Based X-Ray Absorption Techniques
5.8 X-Ray Absorption Near Edge Structure
5.9 Extended X-Ray Absorption Fine Structure
5.10 Conclusions
References
6. Recent Progress on Perovskite-Based Solar Cells
6.1 Introduction
6.2 Device Structure and Working Principle of PSCs
6.3 Perovskite-Based Solar Cells
6.4 Conclusion
References
7. BiFeO3-Based Materials For Augmented Photoactivity
7.1 Introduction
7.1.1 Photocatalytic Water Splitting
7.1.2 Photocatalytic Conversion of CO2
7.1.3 Photocatalytic Fixation of Nitrogen
7.1.4 Selective Organic Transformation for the Synthesis of Fine Chemicals
7.1.5 Photodegradation of Pollutants
7.2 Structure, Physicochemical, and Photocatalytic Activity of BiFeO3
7.3 Elemental Doping in BFO
7.3.1 PXRD Studies
7.3.2 Morphological Studies
7.3.3 XPS Studies
7.3.4 Optical Property Studies
7.3.5 Effect of Doping on Photocatalytic Activity of BFO
7.4 BFO Semiconductor Heterojunction Construction
7.4.1 Heterojunction Construction With Wide Band Gap Semiconductors
7.4.2 Heterojunction Construction With Narrow Band Gap Semiconductors
7.5 Separation Ability and Reproducibility
7.6 Conclusion and Perspectives
7.7 Acknowledgement
References
8. Photocatalytic Degradation of Pollutants Using ZnTiO3-Based Semiconductor
8.1 Introduction
8.2 Synthesis of ZnTiO3
8.3 Fundamental Need and Basic Mechanism for Photocatalytic Degradation of Pollutants
8.4 Photocatalytic Degradation of Pollutants Based on ZnTiO3
8.5 Conclusion
References
9. Types of Perovskite Materials
Abbreviations
9.1 Introduction
9.1.2 Types of Perovskite
9.1.2.1 ABO3 Type of Perovskite Materials
9.1.2.2 Oxygen and Cation-Deficient Perovskites
9.1.2.3 Complex Perovskites
9.1.2.4 Layered Perovskites
9.1.2.4.1 The Dion-Jacobson Series of Layered Perovskites
9.1.2.4.2 Ruddlesden-Popper Phases
9.1.2.4.3 Aurivillius Family
References
10. Effects of Various Additives to CH3NH3PbI3 Perovskite Solar Cells
10.1 Introduction
10.2 Crystal Structures of Perovskite Halides
10.3 Basic Configuration of Solar Cells
10.4 Cl Doping to Perovskites
10.5 Sb or As Doping to Perovskites
10.6 Highly (100)-Oriented Perovskites
10.7 Cu Doping to Perovskites
10.8 K/FA Doping to Perovskites
10.9 Morphology Control by Polysilane
10.10 High-Temperature Annealed Perovskites
10.11 Conclusion
Acknowledgments
References
Index
WILEY END USER LICENSE AGREEMENT
Отрывок из книги
Scrivener Publishing
.....
The ASA one dimensional (1-D) device simulator is very appropriate for simulating thin film structures of silicon solar cells. The program fulfils the usual requirements for simulating thin-film solar cell devices [3].
For modeling silicon thin-film devices the electronic structure of hydrogenated amorphous silicon (a-Si: H) and hydrogenated microcrystalline silicon (μc-Si: H) need to be considered. The atomic structure of a-Si:H shows spatial disorder leading to the energy band gap with edges of conduction band (CB) and valance band (VB) not well-defined with a continuous density of states (DOS). While studying the transport properties of carriers in a-Si:H, in the DOS distribution, it is necessary to differentiate between the localized states and the extended states. Within the mobility gap, the localized states greatly affect the processes of charge trapping followed by recombination. Thus, as is the case with crystalline semiconductor modeling, the charge trapped inside the localized states cannot be neglected. For the calculation of recombination-generation (R-G) statistics different methods are required for the localized states in the mobility gap of a-Si:H as they can be of different nature. The Shockley-Read-Hall (SRH) recombination is not used for amorphous films in device structures in a-Si:H to model recombination as the states introduced by dopants and/or impurities are negligible compared to the recombination via the tail states or dangling bond states. Though, SRH recombination based on the carrier lifetimes can be used for crystalline materials in the ASA program.
.....