Isotopic Constraints on Earth System Processes
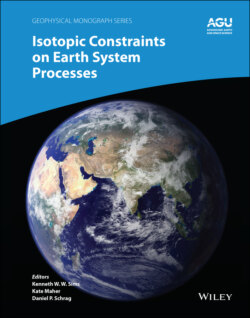
Реклама. ООО «ЛитРес», ИНН: 7719571260.
Оглавление
Группа авторов. Isotopic Constraints on Earth System Processes
Table of Contents
List of Tables
List of Illustrations
Guide
Pages
Geophysical Monograph Series
Geophysical Monograph 273. Isotopic Constraints on Earth System Processes
LIST OF CONTRIBUTORS
PREFACE
PART I: HIGH‐TEMPERATURE/DEEP EARTH PROCESSES
PART II: LOW‐TEMPERATURE/SHALLOW EARTH PROCESSES
REFERENCES
ABOUT THE COMPANION WEBSITE
DEDICATION
1 High‐Temperature Kinetic Isotope Fractionation of Silicate Materials
ABSTRACT
1.1. INTRODUCTION
1.2. DIFFUSION IN MULTI‐COMPONENT CONDENSED SYSTEMS: THEORY AND DEFINITIONS. 1.2.1. Fick’s Laws and the Diffusion Matrix
1.2.2. Effective Binary Diffusion Coefficients
1.2.3. Self‐Diffusion Coefficients
1.2.4. Thermal (Soret) Diffusion Coefficients
1.3. KINETIC ISOTOPE FRACTIONATION DURING DIFFUSION BETWEEN NATURAL MELTS
1.3.1. Laboratory Experiments Documenting Ca Isotope Fractionation by Diffusion Between Molten Rhyolite and Basalt
1.3.2. Isotope Fractionation between Melts from a Natural Setting
1.4. ISOTOPE FRACTIONATION BY SORET DIFFUSION
1.4.1. The Soret Coefficient
1.4.2. Soret Isotope Fractionation in Silicate Liquids
1.5. ISOTOPE FRACTIONATION BY DIFFUSION IN SILICATE MINERALS
1.5.1. Experiments documenting Lithium Isotopic Fractionation by Diffusion in Pyroxene
1.5.2. Natural Examples of Lithium Zoning and Isotopic Fractionation by Diffusion in Pyroxenes
1.5.3. Lithium Isotopic Fractionation by Diffusion in Olivine
1.5.4. Fe‐Mg zoning and Fe and Mg Isotopic Fractionation in Olivine
1.6. ISOTOPE FRACTIONATION BY EVAPORATION FROM SILICATE MELTS
1.6.1. The Hertz‐Knudsen Evaporation Equation
1.6.2. Rayleigh Fractionation
1.6.3. High‐Temperature Vacuum Evaporation Experiments
1.6.4. Evidence of Evaporation in Natural CAIs from Chondritic Meteorites
1.7. SUMMARY
1.8. THOUGHTS ON FURTHER RESEARCH
REFERENCES
2 Ca and K Isotope Fractionation by Diffusion in Molten Silicates: Large Concentration Gradients Are Not Required to Induce Large Diffusive Isotope Effects
ABSTRACT
2.1. INTRODUCTION
2.2. METHODS. 2.2.1. Experiments
2.2.2. Electron Microprobe Analyses
2.2.3. Ca Isotopic Measurements
2.2.4. K Isotopic Measurements
2.3. RESULTS. 2.3.1. Major Element Diffusion Profiles
2.3.2. Ca and K Isotopes
2.4. DISCUSSION
2.5. MODELING
2.5.1. General Multicomponent Diffusion
2.5.2. The Zhang (1993) Modified Effective Binary Diffusion Model
Model Validation and Behavior
Model Applied to the Rhyolite‐Phonolite Couples
Model for the Isotope Ratio Profiles
2.5.3. Comparison to Previous Studies
2.6. CONCLUSIONS AND POSSIBLE FUTURE APPLICATIONS
APPENDIX LINEAR VERSUS EXPONENTIAL DEPENDENCE OF ACTIVITY ON SIO2
ACKNOWLEDGMENTS
REFERENCES
3 Calcium Isotope Constraints on Recycled Carbonates in Subduction‐Related Magmas
ABSTRACT
3.1. INTRODUCTION
3.2. ANALYTICAL METHODS AND SAMPLES. 3.2.1. Double‐spike Thermal Ionization Mass Spectrometry Calcium Isotope Measurements
3.2.2. Igneous Samples Characterized for Calcium Isotope Composition
3.3. RESULTS
3.4. DISCUSSION. 3.4.1. Calcium Isotopic Record of Marine Carbonates
3.4.2. Calcium Isotopic Record of Mantle‐Derived Rocks
3.4.3. Calcium Isotopes Exhibit no Evidence for Carbonate Sediment Recycling at Subduction Zones
3.4.4. Mantle Source(s) of Calcium in Carbonatite Magmas
3.4.5. Origin of the Light Calcium Isotope Composition of Laacher See and other Intrusive Carbonatites
3.5. CONCLUSIONS
ACKNOWLEDGMENTS
REFERENCES
4 Reassessing the Role of Continental Lithospheric Mantle in Cenozoic Magmatism, Southwestern North America
ABSTRACT
4.1. INTRODUCTION
4.2. GEOLOGIC BACKGROUND & GENERAL TERMINOLOGY. 4.2.1. Cenozoic Geologic History of SWNA
4.2.2. Definition of Continental Lithospheric Mantle
4.3. METHODS/DATA
4.4. RESULTS
4.5. DISCUSSION. 4.5.1. Do Nd Isotope Data Support a CLM Source for Mafic Volcanic Rocks in SWNA?
4.5.2. Isotopic Composition of CLM from Xenolith Studies
Comparison of Basalt and Mantle Xenolith Isotopic Compositions
4.5.3. Cenozoic Metasomatism of CLM
Low εNd (T), Low Ta/Th Volcanic Rocks
4.5.4. Physical Evolution of Deep Lithosphere
Destruction of Mantle Lithosphere
4.6. CONCLUSIONS
ACKNOWLEDGMENTS
REFERENCES
5 Rhyolite Ignimbrite Generation in the Northern Andes: The Chalupas Caldera, Ecuador
ABSTRACT
5.1. INTRODUCTION
5.2. GEOLOGICAL SETTING AND AGE OF THE CHALUPAS CALDERA
5.3. GEOCHEMICAL RESULTS. 5.3.1. Analytical Techniques
5.3.2. Major Element Geochemistry
5.3.3. Trace Element Geochemistry
5.3.4. Isotope Geochemistry
5.3.5. Metamorphic Basement Rocks of the Eastern Cordillera
5.4. EVOLUTION OF THE CHALUPAS MAGMATIC SYSTEM. 5.4.1. Role of Fractional Crystallization
5.4.2. The Role of Crustal Assimilation
5.4.3. Modeling Results
5.4.4. Model Discussion
5.4.5. Assimilation and Crustal Thickness
5.5. CRUSTAL STRUCTURE, MAGMA SUPPLY, AND TRANSPORT
5.5.1. Crustal and Magma Density
5.5.2. Temperature Considerations
5.5.3. Subduction Zone Magma Supply and Magmatic Timescales
5.5.4. Timescales of Transport and Assimilation
5.6. CHALUPAS ERUPTION VOLUME AND MAGMA SUPPLY
5.7. SUMMARY AND CONCLUSIONS
APPENDIX 5A Appendix
Pre‐Caldera Lavas
Chalupas Ignimbrite
Post‐Caldera Lavas
Summary of Age Data
APPENDIX 5B MINERAL CHEMISTRY AND PETROGRAPHIC DESCRIPTIONS
5B.1. Pre‐Caldera Lavas
5B.2. Post‐Caldera Lavas
5B.3. Chalupas Ignimbrite
5B.4. Lithics from the Chalupas Ignimbrite
APPENDIX 5C MODELS FOR CRYSTAL FRACTIONATION, ASSIMILATION-FRACTIONAL CRYSTALLIZATION, AND MAGMA FLUXES. 5C.1. Quantitative Estimation of Crystal Fractionation Effects
5C.2. Assimilation‐Fractional Crystallization (AFC) Model Details
5C.3. Relationship between f and Crustal Fraction (fc) in the AFC Model
5C.4. Magma Supply Considerations
5C.5. Magma Supply Requirements
5C.6. Diapir Formation and Transport through the Lower and Mid‐Crust
ACKNOWLEDGMENTS
REFERENCES
6 Xenolith Constraints on “Self‐Assimilation” and the Origin of Low δ18O Values in Mauna Kea Basalts
ABSTRACT
6.1. INTRODUCTION
6.2. SAMPLES AND ANALYTICAL METHODS
6.2.1. EPMA Analysis of Mineral Major Element Composition
6.2.2. Clinopyroxene Trace Element Analysis by LA‐ICP‐MS
6.2.3. Oxygen Isotope Analysis by Laser Fluorination Gas Source Mass Spectrometry
6.2.4. Strontium‐Nd‐Pb Isotope Analysis by TIMS and MC‐ICP‐MS
6.3. RESULTS
6.4. DISCUSSION. 6.4.1. Constraints on Parental Melts of Mauna Kea Xenoliths
6.4.2. Constraints on The P‐T Conditions Of Xenolith Formation and Later Re‐Equilibration
6.4.3. Role of Pacific Crust Assimilation and Edifice Self‐Assimilation
6.4.4. Oxygen Isotope Compositional Variability in the Hawaiian Plume?
6.4.5. Significance of Self‐Assimilation for Interpretation of Geochemical Signatures in Hawaiian Basalts
6.5. CONCLUSIONS
ACKNOWLEDGMENTS
REFERENCES
SUPPLEMENTAL DATA
7 Monitoring Volcanic Activity Through Combined Measurements of CO2 Efflux and (222Rn) and (220Rn) in Soil Gas: An Application to Mount Etna, Italy
ABSTRACT
7.1. INTRODUCTION
7.2. BACKGROUND. 7.2.1. Mt. Etna Volcanic Activity During 2006 to 2009
Phase 1
Phase 2
Phase 3
Phase 4
7.2.2. Prior Work Utilizing Coupled 220Rn/222Rn and CO2 Efflux Measurements on Mt. Etna
7.3. SAMPLING STRATEGY AND ANALYTICAL METHODS. 7.3.1. Sampling Strategy
7.3.2. Soil 222Rn and 220Rn Measurements
7.3.3. Soil CO2 Concentration and Efflux Measurements
7.3.4. Carbon Isotope Measurements
7.4. SYNOPSIS OF THIS STUDY’S RESULTS. 7.4.1. Coupled CO2 Efflux and (220Rn/222Rn)
7.4.2. Carbon Isotopes
7.5. THE SOIL GAS DISEQUILIBRIUM INDEX (SGDI)
7.6. RELATIONSHIP BETWEEN FILTERED SGDI DATA AND VOLCANIC ACTIVITY OF MT. ETNA
7.6.1. Modelling
7.6.2. Comparison of SGDI to Other Monitoring Proxies
7.7. SUMMARY
APPENDIX STATISTICAL TREATMENT OF SGDI DATA. Cluster Analysis and Spatial Distributions
Analysis of SGDI Time Series
Definition of Anomalies in the SGDI Time Series
ACKNOWLEDGMENTS
REFERENCES
8 The Carbon Isotope Record and Earth Surface Oxygenation
ABSTRACT
8.1. INTRODUCTION
8.2. THE CARBON ISOTOPE BUDGET
8.3. forg AND THE OXYGEN BUDGET
8.4. OXYGEN SINKS IN A LOW‐OXYGEN WORLD
8.4.1. Carbon as a Precambrian Oxygen Sink
8.4.2. Sulfur as a Precambrian Oxygen Sink
8.4.3. Iron as a Precambrian Oxygen Sink
8.4.4. Other Precambrian Oxygen Sinks
8.5. RESOLVING THE pO2 – forg PARADOX
8.6. PREDICTIONS OF THE AUTHIGENIC FEEDBACK HYPOTHESIS
8.7. CONCLUSIONS
REFERENCES
9 Detrital Garnet Geochronology: A New Window into Ancient Tectonics and Sedimentary Provenance
ABSTRACT
9.1. INTRODUCTION AND MOTIVATION
9.2. THEORETICAL FEASIBILITY OF DETRITAL GARNET GEOCHRONOLOGY
9.2.1. Age Precision vs. Single Garnet Grain Diameter
9.2.2. Age Accuracy: Blanks
9.2.3. Age Accuracy: Second Point on the Isochron
9.3. DETAILED METHODOLOGY
9.3.1. Sample Processing Prior to Chemical Analysis. Sample Collection and Garnet Separation
Pre‐Rinse Protocol for Surface Contaminant Removal
Major Element Garnet Chemistry and Grain Selection. SEM surface analysis
Grain selection and preparation
9.3.2. Partial Dissolution: Leaching out the Inclusions
Hydrofluoric Acid Partial Dissolution
Perchloric Acid Partial Dissolution
Nitric Acid Partial Dissolution
9.3.3. Full Dissolution of Pure Garnet Residue
9.3.4. Column Chemistry
9.3.5. Thermal Ionization Mass Spectroscopy
9.3.6. Blank Correction
9.3.7. Age Determination
9.4. CASE STUDIES
9.4.1. Preliminary Work: Multi‐Grain Detrital Garnet Ages in Beach Sand from Hampton Beach, New Hampshire
9.4.2. Bulk vs. Detrital Garnet Methodology Test: Townshend Dam, Vermont
Methodology
Results and Interpretation
9.4.3. Single‐Grain Detrital Garnet Ages in Stream Alluvium: Townshend Dam, Vermont
Methodology
Results and Interpretation
9.4.4. Single‐Grain Detrital Garnet in Stream Alluvium: Southern Appalachians
9.4.5. Dating Detrital Garnet in Sedimentary Rocks: Scotland
Methodology
Results and Interpretation
9.5. CONCLUSION
ACKNOWLEDGMENTS
REFERENCES
10 A Review of the Development of Cr, Se, U, Sb, and Te Isotopes as Indicators of Redox Reactions, Contaminant Fate, and Contaminant Transport in Aqueous Systems
ABSTRACT
10.1. INTRODUCTION
10.2. MASS SPECTROMETRY AND SAMPLE PREPARATION METHODS
10.2.1. Mass Spectrometry. Double Spike and Hydride Generation Techniques
Chromium Mass Spectrometry
Selenium Mass Spectrometry
Uranium Mass Spectrometry
Antimony Mass Spectrometry
Tellurium Mass Spectrometry
10.2.2. Sample Preparation. Chromium Sample Preparation
Se Sample Preparation
Uranium Sample Preparation
Antimony and Tellurium Sample Preparation
10.3. CURRENT UNDERSTANDING OF ISOTOPIC FRACTIONATION
10.3.1. Equilibrium Fractionation Theory and Calculations
10.3.2. Kinetic Fractionation
10.3.3. Time Scales for Attainment of Equilibrium
10.3.4. Experimental Determinations of Isotopic Fractionation
Reduction‐Driven Isotopic Fractionation
Oxidation‐Driven Isotopic Fractionation
Adsorption‐Driven Isotopic Fractionation
Volatilization and other Processes
10.4. APPLICATIONS OF CR, U, AND SE ISOTOPE MEASUREMENTS
10.4.1. Chromium Isotopes
10.4.2. Uranium Isotope Applications
10.4.3. Selenium Isotope Applications
10.4.4. Te and Sb Isotope Field Data
10.5. REACTIVE TRANSPORT MODELS: AN ESSENTIAL PART OF THE SCIENCE
10.5.1. Rayleigh Distillation Models and their Limitations
10.5.2. Application to Heavy Isotopes in Laboratory and Natural Environments
10.5.3. Beyond Rayleigh: Heavy Isotopes in Reactive Transport Frameworks
10.6. CONCLUSIONS AND OUTLOOK
APPENDIX
ACKNOWLEDGMENTS
REFERENCES
11 The Effects of Reactive Transport on Sulfur Isotopic Compositions in Natural Environments
ABSTRACT
11.1. MOTIVATION
11.2. SULFUR ISOTOPE INTRODUCTION
11.2.1. Constraining the Sulfur Isotope Fractionation Factor
11.2.2. Variation in the Sulfur Isotope Fractionation Factor
11.2.3. A Case Study
11.3. REACTIVE TRANSPORT EFFECTS ON SULFUR ISOTOPE‐SIGNATURES. 11.3.1. An Example of Marine Sedimentary Profiles
11.3.2. An Example of Aquifers
11.4. SUMMARY
REFERENCES
12 A Reactive Transport Framework Describing Covariation in the Isotopic Ratios of Multiple Elements in Natural Systems
ABSTRACT
12.1. MOTIVATION
12.1.1. Open Systems
12.1.2. Multi‐Isotope Analysis
12.1.3. Multi‐Isotopic Reactive Transport Modeling
12.2. A SYNTHETIC MODEL STUDY OF PAIRED ISOTOPE SIGNATURES
12.2.1. Basic Model Setup
12.2.2. Structurally Heterogeneous Flow Fields
12.2.3. Point Sources
12.2.4. Point Sources with Dilution
12.3. SUMMARY
REFERENCES
13 Stable Ca Isotope Fractionation in Cenozoic Marine Mammals: Beyond Biomineralization and Trophic Positioning
ABSTRACT
13.1. INTRODUCTION
13.2. BACKGROUND. 13.2.1. Stable Ca Isotope Fractionation in Nature
13.2.2. Bowhead Whales: Evolution, Ontogenesis, and Ecology
13.3. METHODS. 13.3.1. Sample Collection
13.3.2. Structural Material Analytical Techniques
13.3.3 Baleen Material Analytical Techniques
13.3.4. Defining the Window of Trophic Discrimination
13.4. RESULTS. 13.4.1. Marine Mammal Bone Material
13.4.2. Bowhead Whale Baleen Material
13.5. DISCUSSION
13.5.1. Fossil Marine Mammal Excursion
13.5.2. Modern Odontocete Excursion
13.5.3. Modern Mysticete Excursion
Variations in Fractionation Factor
Isotopically Heavy Prey
Low Ca Concentrations of Prey
Stable Ca Fractionation During Baleen Growth
Other Anomalous Ca Isotope Measurements
13.6 CONCLUSIONS
APPENDIX 13A MARINE MAMMAL BONE ANALYSIS. 13 A.1. Structural Material Sample Preparation
13 A.2. Sample Purification and Matrix Separation
13 A.3. Sample Introduction and Analysis
APPENDIX 13B BALEEN ANALYSIS I. 13 B.1. Baleen Material Sample Preparation
13 B.2. Sample Purification and Matrix Separation
13 B.3. Sample Introduction and Analysis
13 B.4. Initial Measurement of Sample 2012‐B5
APPENDIX 13C BALEEN ANALYSIS II
ACKNOWLEDGMENTS
REFERENCES
INDEX
WILEY END USER LICENSE AGREEMENT
Отрывок из книги
212 The Early Earth: Accretion and Differentiation James Badro and Michael Walter (Eds.)
.....
270 Muography: Exploring Earth’s Subsurface with Elementary Particles László Oláh, Hiroyuki K. M. Tanaka, and Dezso˝ Varga (Eds.)
271 Remote Sensing of Water‐Related Hazards Ke Zhang, Yang Hong, and Amir AghaKouchak (Eds.)
.....