Wireless RF Energy Transfer in the Massive IoT Era
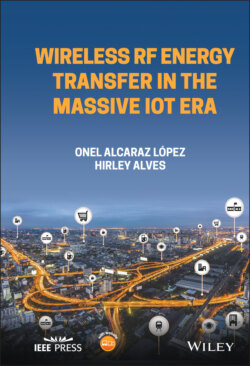
Реклама. ООО «ЛитРес», ИНН: 7719571260.
Оглавление
Hirley Alves. Wireless RF Energy Transfer in the Massive IoT Era
Wireless RF Energy Transfer in the Massive IoT Era. Towards Sustainable Zero-energy Networks
Contents
List of Illustrations
List of Tables
Guide
Pages
Preface. Why We Wrote This Book
Structure of the Book
How to Use This Book
Acknowledgments
Acronyms
Mathematical Notation
About the Companion Website
1 Massive IoT
1.1 Selected Use-cases and Scenarios
1.2 Key Technologies
1.3 Requirements and KPIs
1.4 Key Enablers
1.4.1 Holistic and Globally Scalable Massive IoT
1.4.2 Sustainable Connectivity
1.5 Final Remarks and Discussions
Notes
2 Wireless RF Energy Transfer: An Overview. 2.1 Energy Harvesting
2.1.1 EH Sources
2.1.2 RF Energy Transfer
2.2 RF–EH Performance
Analytical Models
2.2.2 State-of-the-art on RF EH
2.3 RF–EH IoT
2.3.1 Architectures of IoT RF EH Networks
2.3.2 Green WET
2.3.3 WIT-WET Layouts
2.3.4RF EH in IoT Use Cases
2.4 Enabling Efficient RF-WET
2.4.1 Energy Beamforming
2.4.2 CSI-limited Schemes
2.4.3 Distributed Antenna System
2.4.4 Enhancements in Hardware and Medium. Ultra-low-power Receivers
2.4.5 New Spectrum Opportunities
2.4.6 Resource Scheduling and Optimization
2.4.7 Distributed Ledger Technology
2.5 Final Remarks
Notes
3 Ambient RF EH. 3.1 Motivation and Overview
3.1.1 Hybrid of RF–EH and Power Grid
3.1.2 Energy Usage Protocols
3.1.3 On Efficient Ambient RF–RH Designs
3.2 Measurement Campaigns
3.2.1 Greater London (2012)
3.2.2 Diyarbakir (2014)
3.2.3 Flanders (2017-2019)
3.2.4 Other Measurements
3.3 Energy Arrival Modeling
3.3.1 Based on Arbitrary Distributions
3.3.2 Based on Stochastic Geometry
3.4 A Stochastic Geometry-based Study
3.4.1 System Model and Assumptions
3.4.2 Energy Coverage Probability
3.4.3 Average Harvested Energy
3.4.4 Meta-distribution of Harvested Energy
3.4.5 Numerical Results
3.5 Final Considerations
Notes
4 Efficient Schemes for WET. 4.1 EH from Dedicated WET
4.2 Energy Beamforming
Memory-less canonical WET optimization problems
4.2.1 Low-complexity EB Design
4.2.2 CSI-limited Energy Beamforming
4.2.3 Performance Analysis
4.3 CSI-free Multi-antenna Techniques
4.3.1 System Model and Assumptions
4.3.2 Positioning-agnostic CSI-free WET
4.3.3 Positioning-aware CSI-free WET
4.4 On the Massive WET Performance
4.5 Final Considerations
Notes
5 Multi-PB Massive WET. 5.1 On the PBs Deployment
5.1.1 Positioning-aware Deployments
5.1.2 Positioning-agnostic Deployments
5.2 Multi-antenna Energy Beamforming
5.2.1 Centralized Energy Beamforming
5.2.2 Distributed Energy Beamforming
5.2.3 Available RF Energy
5.3 Distributed CSI-free WET
5.3.1 SA, AA–IS and RPS–EMW
5.3.2 Aa–Ss
5.3.3 Rab
5.3.4 Positioning-aware CSI-free Schemes
5.3.5 Numerical Examples
5.4 On the Deployment Costs
Final Remarks
Notes
6 Wireless-powered Communication Networks. 6.1 WPCN Models
6.2 Reliable Single-user WPCN
6.2.1 Harvest-then-transmit (HTT)
6.2.2 Allowing Energy Accumulation
6.2.3 HTT versus FEIPC
6.3 Multi-user Resource Allocation
6.4 Signal Model
6.3.2 Problem Formulation
6.3.3 Optimization Framework
6.3.4 TDMA versus SDMA
6.4 Cognitive MAC
6.4.1 Time Sharing and Scheduling
6.4.2 MAC Protocol at the Device Side
6.4.3 MAC Protocol at the HAP Side
6.5 Final Remarks
Notes
7 Simultaneous Wireless Information and Power Transfer. 7.1 SWIPT Schemes
7.2 Separate EH and ID Receivers
7.2.1 Problem Formulation
7.2.2 Optimal Solution
7.2.3 Performance Results
7.3 Co-located EH and ID Receivers
7.3.1 Time Switching
Problem Formulation
Optimal Solution
7.3.2 Power splitting
7.3.3 TS versus PS
7.4 Enablers for Efficient SWIPT
7.4.1 Waveform Optimization
7.8 Multicarrier SWIPT
7.4.3 Cooperative Relaying
7.4.4 Interference Exploitation
7.4.5 Artificial Intelligence
7.5 Final Considerations
Notes
8 Final Notes
8.1 Summary
8.2 Future Research Directions
Appendix A: A Brief Overview on Finite Block Length Coding
A.1 Finite Block Length Model
Appendix B: Distribution of Transferred RF Energy Under CSI-free WET. B.1 Proof of Theorem 4.2
B.2 Proof of Theorem 4.4
Appendix C: Clustering Algorithms
C.1 Partitioning Methods
C.1.1 K-Means
C.1.2 K-Medoids
C.1.3 K-Modes
C.2 Hierarchical Methods
C.3 Other Methods
C.4 Pre-processing
Appendix D: Required SNR for a Target Decoding Error Probability (Proof of Theorem 6.1)
D.1 On the Convergence of Algorithm 3
Bibliography
Index
WILEY END USER LICENSE AGREEMENT
Отрывок из книги
Onel Alcaraz López
University of Oulu
.....
Even though mMTC is encrusted 5G jargon, it is an umbrella term that specifies the ensemble of solutions toward massive IoT. Thus, it comprises the cellular IoT technologies discussed so far, namely LTE-M and NB-IoT, and solutions under development toward current and next generations, as well as non-cellular technologies, such as LoRa and SigFox. This is because 1. LTE-M and NB-IoT are compliant with the evolution of mobile communications, thus both solutions operate in-band with 5G, and will evolve (so-called future proof) complying with 5G mMTC requirements [9, 25]; 2. mMTC encloses LPWANs; therefore, non-cellular LPWA technologies fall within this definition. Another point is that even with the advantages of cellular IoT, it is unlikely that a single technology will be ubiquitous in a fragmented market. Therefore, future generations beyond 5G are likely to coexist and complement unlicensed solutions as foreseen in [33].
mMTC key challenges are:
.....