Plant Nucleotide Metabolism
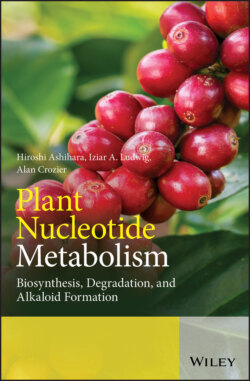
Реклама. ООО «ЛитРес», ИНН: 7719571260.
Оглавление
Hiroshi Ashihara. Plant Nucleotide Metabolism
Table of Contents
List of Tables
List of Illustrations
Guide
Pages
Plant Nucleotide Metabolism – Biosynthesis, Degradation, and Alkaloid Formation
Preface
1 Structures of Nucleotide-Related Compounds. 1.1 Introduction
1.2 Nomenclature and Abbreviations of Nucleotide-Related Compounds
1.3 Chemical Structures of Nucleotide-Related Compounds
1.3.1 Purines
1.3.1.1 Purine Bases
1.3.1.2 Purine Nucleosides
1.3.1.3 Purine Nucleotides
1.3.2 Pyrimidines
1.3.2.1 Pyrimidine Bases
1.3.2.2 Pyrimidine Nucleosides
1.3.2.3 Pyrimidine Nucleotides
1.3.3 Pyridines
1.4 Summary
References
2 Occurrence of Nucleotides and Related Metabolites in Plants. 2.1 Purines and Pyrimidines
2.1.1 Concentration of Purine and Pyrimidine Nucleotides
2.1.2 Concentration of Purine and Pyrimidine Bases and Nucleosides
2.2 Pyridine Nucleotides. 2.2.1 Concentration of Pyridine Nucleotides
2.2.2 Concentration of Nicotinate and Nicotinamide
2.3 Concentration of Cytokinins
2.4 Alkaloids Derived from Nucleotides
2.5 Summary
References
3 General Aspects of Nucleotide Biosynthesis and Interconversions
3.1 Introduction
3.2 De Novo Biosynthesis of Ribonucleoside Monophosphates
3.3 Interconversion of Nucleoside Monophosphates, Nucleoside Diphosphates, and Triphosphates
3.3.1 Nucleoside-Monophosphate Kinase
3.3.2 Specific Nucleoside-Monophosphate Kinases
3.4 Conversion of Nucleoside Diphosphates to Nucleoside Triphosphates
3.4.1 ATP Synthesis by Electron Transfer Systems
3.4.2 Substrate-Level ATP Synthesis
3.4.3 Nucleoside-Diphosphate Kinase
3.5 Biosynthesis of Deoxyribonucleotides
3.6 Nucleic Acid Biosynthesis
3.7 Supply of 5-Phosphoribosyl-1-Pyrophosphate
3.8 Supply of Amino Acids for Nucleotide Biosynthesis
3.9 Nitrogen Metabolism and Amino Acid Biosynthesis in Plants
3.10 Summary
References
4 Purine Nucleotide Biosynthesis De Novo. 4.1 Introduction
4.2 Reactions and Enzymes
4.2.1 Synthesis of Phosphoribosylamine
4.2.2 Synthesis of Glycineamide Ribonucleotide
4.2.3 Synthesis of Formylglycineamide Ribonucleotide
4.2.4 Synthesis of Formylglycinamidine Ribonucleotide
4.2.5 Synthesis of Aminoimidazole Ribonucleotide
4.2.6 Synthesis of Aminoimidazole Carboxylate Ribonucleotide
4.2.7 Synthesis of Aminoimidazole Succinocarboxamide Ribonucleotide
4.2.8 Synthesis of Aminoimidazole Carboxamide Ribonucleotide
4.2.9 Synthesis of IMP via Formamidoimidazole Carboxamide Ribonucleotide
4.2.10 Synthesis of AMP
4.2.11 Synthesis of GMP
4.3 Summary
References
5 Salvage Pathways of Purine Nucleotide Biosynthesis. 5.1 Introduction
5.2 Characteristics of Purine Salvage in Plants
5.3 Properties of Purine Phosphoribosyltransferases
5.3.1 Adenine Phosphoribosyltransferase
5.3.2 Hypoxanthine/Guanine Phosphoribosyltransferase
5.3.3 Xanthine Phosphoribosyltransferase
5.4 Properties of Nucleoside Kinases
5.4.1 Adenosine Kinase
5.4.2 Inosine/Guanosine Kinase
5.4.3 Deoxyribonucleoside Kinases
5.5 Properties of Nucleoside Phosphotransferase
5.6 Role of Purine Salvage in Plants
5.7 Summary
References
6 Interconversion of Purine Nucleotides. 6.1 Introduction
6.2 Deamination Reactions
6.2.1 Routes of Deamination of Adenine Ring
6.2.2 AMP Deaminase
6.2.3 Routes of Deamination of Guanine Ring
6.2.4 Guanosine Deaminase
6.3 Dephosphorylation Reactions
6.4 Glycosidic Bond Cleavage Reactions
6.4.1 Adenosine Nucleosidase
6.4.2 Inosine/Guanosine Nucleosidase
6.4.3 Non-specific Purine Nucleosidases
6.4.4 Recombinant Non-Specific Nucleosidases
6.5 In Situ Metabolism of 14C-Labelled Purine Nucleotides
6.5.1 Metabolism of Adenine Nucleotides
6.5.2 Metabolism of Guanine Nucleotides
6.6 In Situ Metabolism of Purine Nucleosides and Bases
6.6.1 Metabolism of Adenine and Adenosine
6.6.2 Metabolism of Guanine and Guanosine
6.6.3 Metabolism of Hypoxanthine and Inosine
6.6.4 Metabolism of Xanthine and Xanthosine
6.6.5 Metabolism of Deoxyadenosine and Deoxyguanosine
6.7 Summary
References
7 Degradation of Purine Nucleotides. 7.1 Introduction
7.2 (S)-Allantoin Biosynthesis from Xanthine
7.2.1 Xanthine Dehydrogenase
7.2.2 Urate Oxidase
7.2.3 Allantoin Synthase
7.3 Catabolism of (S)-Allantoin
7.3.1 Allantoinase
7.3.2 Allantoate Amidohydrolase
7.3.3 (S)-Ureidoglycine Aminohydrolase
7.3.4 Allantoate Amidinohydrolase
7.3.5 Ureidoglycolate Amidohydrolase
7.3.6 (S)-Ureidoglycolate-urea Lyase
7.3.7 Urease
7.4 Purine Nucleotide Catabolism in Plants
7.5 Accumulation and Utilization of Ureides in Plants. 7.5.1 Ureides in Plant Tissues and Xylem Sap
7.5.2 Role of Ureides in Nitrogen Storage and Transport
7.5.3 Role of Ureides in Germination and Development of Seeds
7.5.4 Ureide Formation in Nodules of Tropical Legumes
7.5.5 Other Role of Ureides in Plants
7.6 Summary
References
8 Pyrimidine Nucleotide Biosynthesis De Novo. 8.1 Introduction
8.2 Reactions and Enzymes of the De Novo Biosynthesis
8.2.1 Synthesis of Carbamoyl-phosphate
8.2.2 Formation of Carbamoyl-aspartate
8.2.3 Formation of Dihydroorotase from Carbamoyl-aspartate
8.2.4 Formation of Orotate from Dihydroorotate
8.2.5 Synthesis of UMP from Orotate
8.2.6 Synthesis of CTP from UTP
8.3 Control Mechanism of De Novo Pyrimidine Ribonucleotide Biosynthesis
8.3.1 Fine Control of the De Novo Pathway
8.3.2 Coarse Control of the De Novo Pathway
8.4 Biosynthesis of Thymidine Nucleotide. 8.4.1 Formation of dUMP
8.4.2 Conversion of UMP to dUMP via dUTP
8.4.3 Conversion of dUMP to dTMP
8.4.4 Thymidine Monophosphate Kinase
8.5 Summary
References
9 Salvage Pathways of Pyrimidine Nucleotide Biosynthesis. 9.1 Introduction
9.2 Characteristics of Pyrimidine Salvage in Plants
9.3 Enzymes of Pyrimidine Salvage
9.3.1 Uracil Phosphoribosyl Transferase
9.3.2 Uridine/Cytidine Kinase
9.3.3 Thymidine Kinase
9.3.4 Deoxyribonucleoside Kinase
9.3.5 Nucleoside Phosphotransferase
9.4 Role of Pyrimidine Salvage in Plants
9.5 Summary
References
10 Interconversion of Pyrimidine Nucleotides. 10.1 Introduction
10.2 Deaminase Reactions
10.2.1 Cytidine Deaminase
10.2.2 Cytosine Deaminase
10.2.3 Deoxycytidylate Deaminase
10.3 Nucleosidase and Phosphorylase Reactions
10.3.1 Uridine Nucleosidase
10.3.2 Thymidine Phosphorylase
10.4 In Situ Metabolism of 14C-Labelled Pyrimidines
10.4.1 Metabolic Fate of Orotate
10.4.2 Metabolic Fate of Uridine and Uracil
10.4.3 Metabolic Fate of Cytidine and Cytosine
10.4.4 Metabolic Fate of Deoxycytidine
10.4.5 Metabolic Fate of Thymidine
10.5 Summary
References
11 Degradation of Pyrimidine Nucleotides. 11.1 Introduction
11.2 Enzymes Involved in the Degradation Routes of Pyrimidines
11.2.1 Dihydropyrimidine Dehydrogenase
11.2.2 Dihydropyrimidinase
11.2.3 β-Ureidopropionase
11.3 The Metabolic Fate of Uracil and Thymine
11.4 Summary
References
12 Growth and Development
12.1 Introduction
12.2 Embryo Maturation
12.3 Germination
12.3.1 Purine Metabolism in Germination
12.3.2 Pyrimidine Metabolism in Germination
12.4 Organogenesis
12.5 Breaking Bud Dormancy
12.6 Fruit Ripening
12.7 Storage Organ Development and Sprouting
12.8 Suspension-Cultured Cells
12.8.1 Nucleotide Pools
12.8.2 Nucleotide Biosynthesis
12.8.3 Nucleotide Availability
12.9 Molecular Studies
12.10 Summary
References
13 Environmental Factors and Nucleotide Metabolism. 13.1 Introduction
13.2 Effect of Phosphate on Nucleotide Metabolism
13.3 Effect of Salts on Nucleotide Metabolism
13.4 Effect of Water Stress
13.5 Effect of Wound Stress
13.6 Effect of Iron Deficiency
13.7 Effect of Light
13.8 Summary
References
14 Occurrence of Purine Alkaloids. 14.1 Introduction
14.2 Chemical Structure of Purine Alkaloids
14.3 Occurrence of Purine Alkaloids in Plants
14.3.1 Purine Alkaloids in Tea and Related Species
14.3.2 Purine Alkaloids in Coffee and Related Species
14.3.3 Purine Alkaloids in Maté
14.3.4 Purine Alkaloids in Cacao and Related Species
14.3.5 Purine Alkaloids in Cola Species
14.3.6 Purine Alkaloids in Guaraná and Related Species
14.3.7 Purine Alkaloids in Citrus Species
14.3.8 Purine Alkaloids in Other Plants
14.4 Summary
References
15 Biosynthesis of Purine Alkaloids. 15.1 Introduction
15.2 A Brief History of Caffeine Biosynthesis Research
15.3 Caffeine Biosynthesis Pathway
15.3.1 N-Methyltransferase Nomenclature
15.3.2 Formation of 7-Methylxanthine from Xanthosine
15.3.3 7-Methylxanthosine Synthase
15.3.4 N-Methylnucleosidase
15.3.5 Formation of Caffeine from 7-Methylxanthine
15.3.6 Caffeine Synthase
15.3.7 Theobromine Synthase
15.4 Genes and Proteins of Caffeine Synthase Family
15.5 Xanthosine Biosynthesis from Purine Nucleotides
15.5.1 De Novo Purine Route
15.5.2 Adenosine Monophosphate Route
15.5.3 S-Adenosyl-L-methionine Cycle Route
15.5.4 Nicotinamide Adenine Diphosphate Catabolism Route
15.5.5 Guanosine Monophosphate Route
15.6 Summary
References
16 Physiological and Ecological Aspects of Purine Alkaloid Biosynthesis. 16.1 Introduction
16.2 Physiology of Caffeine Biosynthesis
16.2.1 Purine Alkaloid Biosynthesis in Different Species
16.2.2 Camellia
16.2.3 Coffea
16.2.4 Theobroma
16.2.5 Maté
16.2.6 Guaraná
16.2.7 Citrus
16.3 Subcellular Localization of Caffeine Biosynthesis
16.3.1 Caffeine Synthase
16.3.2 The De Novo Route Enzymes
16.3.3 The AMP Route Enzymes
16.3.4 The SAM Route Enzymes
16.3.5 Subcellular Localization and Transport of Intermediates
16.4 Regulation of Caffeine Biosynthesis
16.5 Ecological Roles of Caffeine
16.5.1 Allelopathic Function Theory
16.5.2 Effect of Caffeine on Plant Growth
16.5.3 Allelopathy in Natural Ecosystems
16.5.4 Chemical Defence Theory
16.6 Summary
References
17 Metabolism of Purine Alkaloids and Biotechnology. 17.1 Introduction
17.2 Metabolism of Purine Alkaloids. 17.2.1 Methylurate Biosynthesis
17.2.2 The Major Pathway of Caffeine Degradation
17.2.3 Purine Catabolic Pathways in Alkaloid Plants
17.3 Diversity of Purine Alkaloid Metabolism in Plants. 17.3.1 Coffea Species
17.3.2 Camellia Species
17.3.3 Maté Species
17.3.4 Cacao Species
17.3.5 Other Plant Species
17.3.6 Bacteria
17.4 Biotechnology of Purine Alkaloids
17.4.1 Decaffeinated Coffee Plants
17.4.2 Decaffeinated Tea Plants
17.5 Caffeine-Producing Transgenic Plants
17.5.1 Antiherbivore Activity
17.5.2 Antipathogen Activity
17.6 Summary
References
18 Pyridine (Nicotinamide Adenine) Nucleotide Biosynthesis De Novo. 18.1 Introduction
18.2 Two Distinct Pathways of De Novo Nicotinate Mononucleotide Biosynthesis
18.3 The Outline of the De Novo Pathway of NAD Biosynthesis in Plants
18.4 Enzymes Involved in De Novo NAD Synthesis in Plants
18.4.1 L-Aspartate Oxidase and Quinolinate Synthase
18.4.2 Quinolinate Phosphoribosyltransferase
18.4.3 Nicotinate Mononucleotide Adenylyltransferase
18.4.4 NAD Synthetase
18.4.5 NAD Kinase
18.5 Summary
References
19 Pyridine Nucleotide Cycle. 19.1 Introduction
19.2 Pyridine Nucleotide Cycle
19.2.1 Major Pyridine Nucleotide Cycles in Plants
19.2.2 Alternative Pyridine Nucleotide Cycles in Plants
19.2.3 Rate-Limiting Step of the Pyridine Cycle
19.3 Catabolism of NAD. 19.3.1 Reactions from NAD to Nicotinate
19.3.2 Degradation of Pyrimidine Ring
19.3.3 Nicotinate Conversion to Nicotinate-N-Glucoside and N-Methylnicotinate
19.4 Enzymes Involved in NAD Catabolism. 19.4.1 Direct NAD Cleavage Enzymes
19.4.2 NAD Pyrophosphatase
19.4.3 5′-Nucleotidase and Nicotinamide Riboside Nucleosidase
19.4.4 Nicotinamidase and Nicotinamide Riboside Deaminase
19.5 Salvage of Nicotinamide and Nicotinate
19.5.1 Nicotinate Phosphoribosyltransferase
19.5.2 Nicotinate Riboside Kinase
19.6 Summary
References
20 Occurrence and Biosynthesis of Pyridine Alkaloids. 20.1 Introduction
20.2 Occurrence of Pyridine Alkaloids. 20.2.1 Trigonelline in Plants
20.2.2 Other Pyridine Alkaloids in Plants
20.3 Biosynthesis of Pyridine Alkaloids
20.3.1 Trigonelline Biosynthesis
20.3.2 Nicotinate N-Glucoside Biosynthesis
20.3.3 The Diversity of Biosynthetic Reactions
20.3.3.1 Ferns
20.3.3.2 Gymnosperms
20.3.3.3 Angiosperms
20.3.3.4 Nicotinate Conjugate Formation
20.3.4 Biosynthesis of Ricinine
20.3.5 Biosynthesis of Nicotine (Pyridine Ring)
20.4 Summary
References
21 Physiological Aspect and Biotechnology of Trigonelline. 21.1 Introduction
21.2 Physiological Aspect of Trigonelline Biosynthesis. 21.2.1 Coffee
21.2.2 Leguminous Plants
21.3 Physiological Aspects of Nicotinate N-Glucoside Biosynthesis
21.4 The Role of Trigonelline in Plants
21.4.1 Role of Trigonelline as a Nutrient Source
21.4.2 Role of Trigonelline as a Compatible Solute
21.4.3 Trigonelline and Nyctinasty
21.4.4 Cell Cycle Regulation
21.4.5 Detoxification of Nicotinate
21.4.6 Signal Transduction
21.4.7 Role of Host Selection by Herbivores
21.5 Biotechnology of Trigonelline
21.6 Summary
References
22 Sugar Nucleotides. 22.1 Introduction
22.2 The Sugar Nucleotide Moiety
22.3 Enzymes of Sugar Nucleotide Biosynthesis
22.3.1 UDP-Glucose Pyrophosphorylase
22.3.2 UDP-Sugar Pyrophosphorylase
22.3.3 Sucrose Synthase
22.4 Localization of UDP-Glucose-Producing Enzymes
22.5 UDP-Glucose-Interconversion
22.6 Other Metabolites
22.6.1 Cyclic Nucleotides
22.6.2 Diadenosine Tetraphosphate
22.6.3 Purine Alkaloid Glucosides
22.7 Summary
References
23 Cytokinins. 23.1 Introduction
23.2 Adenosine Phosphate-Isopentenyl Formation
23.3 trans-Zeatin Phosphate Synthesis
23.4 Formation of Cytokinin Bases
23.5 Effect of Nucleotide Enzymes in Cytokinins. 23.5.1 Cytokinin Inactivation by Adenine Phosphoribosyltransferase
23.5.2 Homeostasis of Cytokinin by Adenosine Kinase
23.5.3 Endodormancy of Potato and Purine Nucleoside Phosphorylase
23.6 New Purine-Related Plant Growth Regulators
23.7 Summary
References
24 Bioavailability and Potential Impact on Human Health of Caffeine, Theobromine, and Trigonelline. 24.1 Caffeine. 24.1.1 Dietary Caffeine
24.1.2 Bioavailability and Bioactivity of Caffeine
24.2 Theobromine. 24.2.1 Interactions with Flavan-3-ols
24.2.2 Toxicity of Theobromine
24.3 Trigonelline. 24.3.1 Dietary Trigonelline
24.3.2 Bioavailability and Bioactivity of Trigonelline
24.4 Summary
References
Index
WILEY END USER LICENSE AGREEMENT
Отрывок из книги
Hiroshi Ashihara
Ochanomizu University
.....
Biosynthesis of RNA is catalysed by RNA polymerase (DNA-directed RNA polymerase, EC 2.7.7.6). The reaction is:
RNA polymerase, locally, opens the double-stranded DNA (usually about four turns of the double helix) so that one strand of the exposed nucleotides can be used as a template for the synthesis of RNA, namely transcription. A transcription factor and its associated transcription mediator complex must be attached to a DNA binding site, a promoter region, before RNA polymerase can initiate the DNA unwinding at that position. RNA polymerase has intrinsic helicase activity, therefore, no additional enzyme is required to unwind the DNA, in contrast to DNA polymerase. RNA polymerase, not only initiates RNA transcription, it also guides the nucleotides into position, facilitates attachment and elongation, has intrinsic proof reading and replacement capabilities, and a termination recognition function. In eukaryotes, RNA polymerase can build chains as long as 2.4 million nucleotides.
.....