Introduction to Ore-Forming Processes
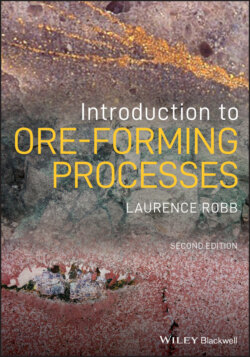
Реклама. ООО «ЛитРес», ИНН: 7719571260.
Оглавление
Laurence Robb. Introduction to Ore-Forming Processes
Table of Contents
List of Tables
List of Illustrations
Guide
Pages
Introduction to Ore‐Forming Processes
Copyright
Preface to the 2nd Edition
Preface to the 1st Edition
Introduction: Mineral Resources. Topics
Introduction and Aims
A Classification Scheme for Ore Deposits
What Makes a Viable Mineral Deposit?
Mineral Resources and Ore Reserves
Some Useful Definitions and Compilations. General Definitions
Periodic Table of the Elements
Common Ore and Gangue Minerals
Native Elements
Metals
Non‐metals
Halides
Sulfides and Sulfosalts
Sulfides
Sulfosalts
Oxides and Hydroxides
Oxides
Hydroxides (or Oxyhydroxides)
Oxysalts
Carbonates
Tungstates
Sulfates
Phosphates
Vanadates
Silicates
Tekto (framework)
Neso (ortho)
Cyclo (ring)
Soro (di)
Phyllo (sheet)
Ino (chain)
Unknown Structure
Geological Time Scale
Natural Resources, Sustainability, and the Environment
Mining and Environmental Responsibility
Summary
Further Reading
1 Igneous Ore‐Forming Processes. Topics
Case Studies
1.1 Introduction
1.2 Magmas and Metallogeny
1.2.1 Crustal Architecture and Mineral Wealth
1.2.2 Magma Types and Metal Contents
1.2.2.1 Basalt
1.2.2.2 Andesite
1.2.2.3 Rhyolite
1.2.2.4 Alkaline Magmas, Carbonatite and Kimberlite
1.3 Why Are Some Magmas More Fertile than Others? The “Inheritance Factor”
1.3.1 The “Late Veneer” Hypothesis of Siderophile Metal Concentration – An Extraterrestrial Origin for Au and Pt?
1.3.2 Diamonds and the Story They Tell
Box 1.1 Diamondiferous Kimberlites and Lamproites: The Orapa Diamond Mine, Botswana and the Argyle Diamond Mine, Western Australia
Argyle
Orapa
1.3.3 Metal Concentrations in Metasomatized Mantle and Their Transfer into the Crust
1.3.4 Metal Enrichment in Carbonatitic and Peralkaline Magmas
Box 1.2 Carbonatites and Alkaline Intrusions as Sources of Critical Metals. Critical Metals
Barreiro (also known as Araxá), Minas Gerais, Brazil
Bayan Obo, China
Why are Alkaline and Carbonatitic Magmas So Fertile?
1.3.5 I‐ and S‐Type Granite Magmas and Metal Specificity
1.4 Partial Melting and Crystal Fractionation as Ore‐Forming Processes
1.4.1 Partial Melting
1.4.1.1 Trace Element Distribution During Partial Melting
1.4.2 Crystallization of Magma
Box 1.3 Partial Melting and Concentration of Incompatible Elements: The Rössing Uranium Deposit
1.4.2.1 The Form and Internal Zonation of Igneous Bodies
Mafic Intrusions
Felsic Intrusions
1.4.2.2 Trace Element Distribution During Fractional Crystallization
Box 1.4 Boundary Layer Differentiation in Granites and Incompatible Element Concentration: The Zaaiplaats Tin Deposit, Bushveld Complex
1.4.3 Fractional Crystallization and the Formation of Monomineralic Chromitite Layers
1.4.3.1 The Irvine Model
Box 1.5 Crystal Fractionation and Formation of Monomineralic Chromitite Layers: The UG1 Chromitite Seam, Bushveld Complex
1.4.3.2 Other Mechanisms for the Formation of Chromitite Layers or Pods
1.4.4 Filter Pressing as a Process of Crystal Fractionation
1.4.4.1 Anorthosite Hosted Ti–Fe Deposits
1.5 Liquid Immiscibility as an Ore‐Forming Process
1.5.1 Silicate–Oxide Immiscibility
1.5.2 Silicate–Sulfide Immiscibility
1.6 A More Detailed Consideration of Mineralization Processes in Mafic Magmas. 1.6.1 A Closer Look at Sulfide Solubility
1.6.2 Sulfide–Silicate Partition Coefficients
1.6.3 The R Factor and Concentration of Low Abundance Trace Elements
1.6.4 Factors that Promote Sulfide Saturation
1.6.4.1 Addition of Externally Derived Sulfur
1.6.4.2 Fractional Crystallization
1.6.4.3 Injection of a New Magma and Magma Mixing
Box 1.6 Addition of External Sulfur and Sulfide Immiscibility: The Komatiite‐Hosted Ni–Cu Deposits at Kambalda, Western Australia
Box 1.7 New Magma Injection and Magma Mixing: The Merensky Reef, Bushveld Complex
Box 1.8 Magma Contamination and Sulfide Immiscibility: The Sudbury Ni–Cu Deposits
1.6.4.4 Magma Contamination
1.6.5 Other Models for Mineralization in Layered Mafic Intrusions
1.6.5.1 PGE Clusters
1.6.5.2 The Role of Chromite in PGE Concentration
1.6.5.3 Hiatus Models
1.6.5.4 Fluid‐Related Infiltration of PGE
1.7 A Model for Mineralization in Layered Mafic Intrusions
1.8 Summary
Further Reading
Note
2 Magmatic‐Hydrothermal Ore‐Forming Processes. Topics
Case Studies
2.1 Introduction
2.2 Some Physical and Chemical Properties of Water
2.3 Formation of a Magmatic Aqueous Phase. 2.3.1 Magmatic Water – Where Does It Come from?
2.3.2 H2O Solubility in Silicate Magmas
2.3.3 The Burnham Model
2.3.3.1 A Note on the Mechanical Effects of Boiling
2.4 The Composition and Characteristics of Magmatic‐ Hydrothermal Solutions
2.4.1 Quartz Veins – What Do They Tell Us About Fluid Compositions?
2.4.2 Major Elements in Magmatic Aqueous Solutions
2.4.3 Other Important Components of Magmatic Aqueous Solutions
2.4.3.1 Magmatic Fluid Compositions from Fluid Inclusion Analysis
2.4.4 Carbon Dioxide in Magmatic Fluids
2.4.5 Other Important Features of Magmatic Fluids
2.5 A Note on Pegmatites and Their Significance to Granite‐ Related Ore‐Forming Processes
2.5.1 Early Models of Pegmatite Genesis
2.5.2 More Recent Ideas on the Origin of Pegmatites
2.6 Fluid–Melt Trace Element Partitioning
2.6.1 Early Experiments on Metal Solubilities in Aqueous Solution
2.6.2 A More Detailed Look at Fluid–Melt Partitioning of Metals
2.6.2.1 Fluid–Melt Partitioning During “First Boiling”
2.6.2.2 Fluid–Melt Partitioning During “Second Boiling”
2.6.2.3 Partitioning of Metals into H2O‐Vapor
2.6.3 Partitioning of Cu, Mo, and W Between Melt and H2O‐Fluid
2.7 Water Content and Depth of Emplacement of Granites – Relationships to Ore‐Forming Processes
2.8 Models for the Formation of Porphyry‐Type Cu, Mo, and W Deposits
2.8.1 The Origin of Porphyry Cu–(Mo) and Porphyry Mo–(Cu) Type Deposits
Box 2.1 Magmatic‐Hydrothermal Fluids Associated with Granite Intrusions (1) – La Escondida Porphyry Copper Deposit, Chile
2.8.2 The Origin of Porphyry W (±Sn) Type Deposits
2.8.3 The Role of Sulfur in the Formation of Porphyry Copper Deposits
2.8.3.1 The Role of Sulfur in Concentrating Metals in Porphyry Systems
2.8.3.2 The Role of Sulfur in Precipitating Ore Minerals in Porphyry Systems
2.9 Near‐Surface Magmatic‐Hydrothermal Processes – The “Epithermal” Family of Au–Ag–(Cu) Deposits
2.9.1 Gold Precipitation Mechanisms in Epithermal Deposits
Box 2.2 Magmatic‐Hydrothermal Processes in Volcanic Environments – the Kasuga and Hishikari Epithermal Au–Ag Deposits, Kyushu, Japan
Kasuga
Hishikari
2.10 Skarn Deposits
Box 2.3 Magmatic‐Hydrothermal Fluids Associated with Granite Intrusions (2) – The MacTung Tungsten Skarn Deposit, Yukon, Canada
2.10.1 Prograde – Isochemical Contact Metamorphism
2.10.2 Prograde – Metasomatism and Replacement
2.10.3 Retrograde – Meteoric Fluid Influx and Main Metal Precipitation
2.11 Fluid Flow in and Around Granite Plutons
Box 2.4 Fluid Flow in and Around Granite Intrusions – Polymetallic Mineralization Associated with the Granites of Cornwall, Southwest England
2.12 The Role of Hydrothermal Fluids in Mineralized Mafic Rocks
2.12.1 The Effects of a Magmatic‐Hydrothermal Fluid on PGE Mineralization in the Bushveld Complex
2.13 Summary
Further Reading
Note
3 Hydrothermal Ore‐Forming Processes. Topics
Case Studies
3.1 Introduction
3.2 Other Fluids in the Earth's Crust and Their Origins
3.2.1 Sea Water
3.2.2 Meteoric Water
3.2.3 Basinal (or Connate) Water
3.2.4 Metamorphic Water
3.2.5 Waters of Mixed Origin
3.3 The Movement of Hydrothermal Fluids in the Earth's Crust
3.3.1 Factors Affecting Fluid Flow at a Crustal Scale
3.3.2 A Note on Hydrostatic Versus Lithostatic Pressure Gradients
3.3.3 Deformation and Hydrothermal Fluid Flow
3.3.4 Other Factors Affecting Fluid Flow and Mineral Precipitation
3.3.4.1 How Do We Know that a Fluid Has Passed Through a Rock?
3.4 Additional Factors Affecting Metal Solubility
3.4.1 The Important Metal–Ligand Complexes in Hydrothermal Solutions
3.4.1.1 Hard Metals
3.4.1.2 Borderline Metals
3.4.1.3 Soft Metals
3.4.2 More on Metal Solubilities in the Aqueous Vapor Phase
3.4.3 A Brief Note on Metal–Organic Complexes
3.5 Precipitation Mechanisms for Metals in Solution
3.5.1 Physico‐Chemical Factors Affecting Metal Precipitation
3.5.1.1 Temperature
3.5.1.2 Pressure
3.5.1.3 Phase Separation (Boiling and Effervescence)
3.5.1.4 Fluid Mixing/Dilution
Box 3.1 Fluid Mixing and Metal Precipitation: The Olympic Dam Iron Oxide–Copper–Gold (IOCG) Deposit, South Australia
3.5.1.5 Fluid/Rock Reactions (pH and Eh Controls)
3.5.2 Adsorption
3.5.3 Biologically Mediated Processes of Metal Precipitation
3.5.3.1 Biomineralization
3.6 Fluid–Rock Interaction – Introduction to Hydrothermal Alteration
3.6.1 Types of Alteration and Their Ore Associations
3.6.1.1 Potassic Alteration
Box 3.2 Alteration and Metal Precipitation: Golden Mile, Kalgoorlie, Western Australia – An Archean Orogenic Gold Deposit
3.6.1.2 Phyllic (or Sericitic) Alteration
3.6.1.3 Propylitic Alteration
3.6.1.4 Argillic Alteration
3.6.1.5 Silication
3.6.1.6 Silicification
3.6.1.7 Carbonatization
3.6.1.8 Greisenization
3.6.1.9 Hematitization
3.7 Metal Zoning and Paragenetic Sequence
3.7.1 Replacement Processes
3.8 Modern Analogues of Ore‐Forming Processes – The VMS–SEDEX Continuum
3.8.1 “Black Smokers” – A Modern Analogue for VMS Deposit Formation
Box 3.3 Exhalative Venting and “Black Smokers” on the Sea Floor: The VMS Deposits of the Troodos Ophiolite Complex, Cyprus
3.8.2 The Salton Sea and Red Sea Geothermal Systems – Modern Analogues for SEDEX Mineralization Processes
3.8.2.1 Salton Sea Geothermal System
3.8.2.2 The Red Sea and the VMS–SEDEX Continuum
Box 3.4 Sedimentary Exhalative (SEDEX) Processes: The Red Dog Zn–Pb–Ag Deposit, Alaska
3.9 Mineral Deposits Associated with Aqueo‐Carbonic Metamorphic Fluids
3.9.1 Orogenic Gold Deposits
3.9.1.1 Archean
3.9.1.2 Proterozoic
3.9.1.3 Phanerozoic
3.9.2 Carlin‐Type Gold Deposits
Box 3.5 Circulation of Orogeny‐Driven Aqueo‐Carbonic Fluids: Twin Creeks – A Carlin‐Type Gold Deposit, Nevada
3.9.3 Quartz Pebble Conglomerate Hosted Gold Deposits
3.10 Ore Deposits Associated with Basinal Fluids
3.10.1 Stratiform Sediment‐Hosted Copper (SSC) Deposits
3.10.2 Mississippi Valley Type (MVT) Pb–Zn Deposits
Box 3.6 Circulation of Sediment‐Hosted Basinal Fluids: 1 The Central African Copperbelt
Box 3.7 Circulation of Sediment‐Hosted Basinal Fluids: 2 The Viburnum Trend, Missouri
3.11 Ore Deposits Associated with Near Surface Meteoric Fluids (Groundwater)
3.11.1 A Brief Note on the Aqueous Transport and Deposition of Uranium
3.11.2 Sandstone‐Hosted Uranium Deposits
3.11.2.1 Colorado Plateau (Tabular) Uranium–Vanadium Type
3.11.2.2 Roll‐Front Type
3.12 Summary
Further Reading
4 Surficial and Supergene Ore‐Forming Processes. Topics
Case Studies
4.1 Introduction
4.2 Principles of Chemical Weathering
4.2.1 Dissolution and Hydration
4.2.2 Hydrolysis and Acid Hydrolysis
4.2.3 Oxidation
4.2.4 Cation Exchange
4.3 Lateritic Deposits
4.3.1 Laterite Formation
4.3.2 Bauxite Ore Formation
Box 4.1 Lateritization: Bauxite – The Los Pijiguaos Deposit, Venezuela
4.3.3 Nickel Laterites
4.3.4 Gold in Laterites
4.3.5 A Note on Platinum Group Element (PGE) Enrichment in Laterites
4.4 Clay Deposits
4.4.1 The Kaolinite (China Clay) Deposits of Cornwall
4.4.2 “Ion‐Adsorption” Rare Earth Element (REE) Deposits in Clays
4.5 Calcrete‐Hosted Deposits
4.5.1 Calcrete‐Hosted or Surficial Uranium Deposits
4.6 Supergene Enrichment of Cu and Other Metals in the Near Surface Environment
4.6.1 Supergene Oxidation of Copper Deposits
4.6.1.1 A Note on Supergene Enrichment of Other Metals
Box 4.2 Supergene Processes: Supergene and “Exotic” Mineralization in Porphyry Copper Deposits
4.7 Summary
Further Reading
5 Sedimentary Ore‐Forming Processes. Topics
Case Studies
5.1 Introduction
5.2 Clastic Sedimentation and Heavy Mineral Concentration – Placer Deposits
5.2.1 Basic Principles
5.2.2 Hydraulic Sorting Mechanisms Relevant to Placer Formation
5.2.2.1 Settling
5.2.2.2 Entrainment
5.2.2.3 Shear Sorting
5.2.2.4 Transport Sorting
5.2.3 Application of Sorting Principles to Placer Deposits
5.2.3.1 Small Scale
5.2.3.2 Intermediate Scale
5.2.3.3 Large Scale
5.2.4 A Note Concerning Sediment Sorting in Beach and Eolian Environments
Box 5.1 Placer Processes: The Alluvial Diamond Deposits of the Orange River, Southern Africa
5.2.4.1 Beaches
5.2.4.2 Wind‐Borne Sediment Transport
5.2.5 Numerical Simulation of Placer Processes
5.3 Chemical Sedimentation – Iron‐Formations, Phosphorites, and Evaporites
5.3.1 Iron‐Formations and Ironstones
5.3.1.1 Bog Iron Ores
5.3.1.2 Phanerozoic Ooidal Ironstone (POI) Deposits
5.3.1.3 Banded and Granular Iron‐Formation – An Enigmatic Rock Type
5.3.1.4 Mechanisms by Which BIFs Are Deposited
5.3.1.5 The Periodicity of Iron‐Formation Deposition
5.3.1.6 Transformation of BIFs into Viable Iron Ore Deposits
Box 5.2 Chemical Sedimentation – Banded Iron‐Formations: The Mount Whaleback Iron Ore Deposit, Hamersley Province, Western Australia
5.3.2 Bedded Manganese Deposits
5.3.3 A Note on Ocean Floor Manganese Nodules
5.3.4 Phosphorites
5.3.4.1 A Model for Phosphogenesis Based on Present Day Deposition
5.3.5 Black Shales
5.3.6 Evaporites
Box 5.3 Evaporite Deposits: The Boulby Mine (Na and K) in Cleveland, UK and Salar de Atacama (K and Li) in Chile. Boulby
Salar de Atacama
5.4 Fossil Fuels – Oil/Gas Formation and Coalification
5.4.1 Basic Principles
5.4.2 Oil and Gas Formation (Conventional)
5.4.2.1 Source Rock Considerations and Organic Maturation
5.4.2.2 Petroleum Migration and Reservoir Considerations
5.4.2.3 Entrapment of Oil and Gas
5.4.3 Coalification Processes
Box 5.4 Fossil Fuels – Oil and Gas: The Arabian (Persian) Gulf, Middle East
5.4.3.1 Coal Characteristics
5.4.3.2 A Note Concerning Formation of Economically Viable Coals
5.4.4 Unconventional Hydrocarbons – Shale Gas, Oil Shales, and Tar Sands
5.4.4.1 Shale Gas and Oil Shales
5.4.4.2 Tar Sands (or Oil Sands)
5.4.5 Gas Hydrates
5.5 Summary
Further Reading
Sedimentology and Placer Processes
Chemical Sedimentation and Ore Formation
Fossil Fuels
Note
6 Ore Deposits in a Global Tectonic Context. Topics
6.1 Introduction
6.2 Patterns in the Distribution of Mineral Deposits
6.3 Continental Growth and the Supercontinent Cycle. 6.3.1 Estimations of Continental Growth Rates
6.3.2 Supercontinent Cycles
6.3.2.1 Kenorland
6.3.2.2 Nuna (also referred to as Columbia)
6.3.2.3 Rodinia
6.3.2.4 Pangea
6.4 Geological Processes and Metallogenesis
6.4.1 Evolution of the Hydrosphere and Atmosphere
6.4.2 Secular Decrease in Global Heat Production and Mantle Temperature
6.4.3 Long‐Term Global Tectonic Trends and Mantle Convection
6.4.4 Eustatic Sea Level Changes and “Continental Freeboard”
6.5 Metallogeny Through Time
6.5.1 The Archean Eon
6.5.1.1 The Hadean (>4000 Ma) and Eoarchean (>3600 Ma) stages
6.5.1.2 The Paleo‐, Meso‐, and Neoarchean stages (3600–2500 Ma)
6.5.1.3 Shield formation (pre‐3100 Ma)
6.5.1.4 Cratonization (c. 3100–2500 Ma)
6.5.2 The Proterozoic Eon
6.5.2.1 The Paleoproterozoic Era (2500–1600 Ma)
6.5.2.2 The Mesoproterozoic Era (1600–1000 Ma)
6.5.2.3 The Neoproterozoic Era (1000–541 Ma)
6.5.3 The Phanerozoic Eon
6.5.3.1 Phanerozoic Tectonic Cycles and Metallogeny
6.5.3.2 Time‐Bound and Regional Aspects of Phanerozoic Metallogeny
6.6 Plate Tectonic Settings and Ore Deposits – A Summary
6.6.1 Extensional Settings
6.6.2 Compressional Settings
6.7 Summary
Further Reading
References
Index
WILEY END USER LICENSE AGREEMENT
Отрывок из книги
Laurence Robb University of Oxford Oxford, UK
Second Edition
.....
Figure 3 Simplified scheme illustrating the conceptual difference between mineral resources and ore reserves as applied to mineral occurrences. The scheme forms the basis for a more unified description of ore deposits as now required in terms of legislation that has been passed in most major mineral producing jurisdictions.
This section is not intended to provide a comprehensive glossary of terms used in this book. There are, however, several terms that are used throughout the text where a definition is either useful or necessary in order to avoid ambiguity. The following definitions are consistent with those provided in the Glossary of Geology (Bates and Jackson 1987) and The Encyclopedia of the Solid Earth Sciences (Kearey 1993).
.....