Power Electronics-Enabled Autonomous Power Systems
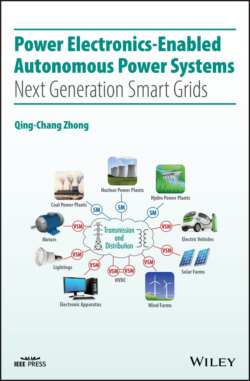
Реклама. ООО «ЛитРес», ИНН: 7719571260.
Оглавление
Qing-Chang Zhong. Power Electronics-Enabled Autonomous Power Systems
Table of Contents
List of Tables
List of Illustrations
Guide
Pages
Power Electronics-Enabled Autonomous Power Systems
List of Figures
List of Tables
Foreword
Preface
Acknowledgments
About the Author
List of Abbreviations
Chapter 1 Introduction. 1.1 Motivation and Purpose
1.2 Outline of the Book
1.3 Evolution of Power Systems
1.3.1 Today's Grids
1.3.2 Smart Grids
1.3.3 Next‐Generation Smart Grids
1.4 Summary
Chapter 2 Synchronized and Democratized (SYNDEM) Smart Grid
2.1 The SYNDEM Concept
2.2 SYNDEM Rule of Law – Synchronization Mechanism of Synchronous Machines
2.3 SYNDEM Legal Equality – Homogenizing Heterogeneous Players as Virtual Synchronous Machines (VSM)
2.4 SYNDEM Grid Architecture. 2.4.1 Architecture of Electrical Systems
2.4.2 Overall Architecture
2.4.3 Typical Scenarios
2.4.3.1 Home Grid
2.4.3.2 Neighborhood Grid
2.4.3.3 Community Grid
2.4.3.4 District Grid
2.4.3.5 Regional Grid
2.5 Potential Benefits
2.6 Brief Description of Technical Routes. 2.6.1 The First‐Generation (1G) VSM
2.6.2 The Second‐Generation (2G) VSM
2.6.3 The Third‐Generation (3G) VSM
2.7 Primary Frequency Response (PFR) in a SYNDEM Smart Grid
2.7.1 PFR from both Generators and Loads
2.7.2 Droop
2.7.3 Fast Action Without Delay
2.7.4 Reconfigurable Virtual Inertia
2.7.5 Continuous PFR
2.8 SYNDEM Roots. 2.8.1 SYNDEM and Taoism
2.8.2 SYNDEM and Chinese History
2.9 Summary
Notes
Chapter 3 Ghost Power Theory
3.1 Introduction
3.2 Ghost Operator, Ghost Signal, and Ghost System. 3.2.1 The Ghost Operator. Definition 1
Lemma 2
Proof:
3.2.2 The Ghost Signal
Lemma 3
Proof:
3.2.3 The Ghost System
Definition 4
Lemma 5
Proof:
Remark 6
3.3 Physical Meaning of Reactive Power in Electrical Systems
3.4 Extension to Complete the Electrical‐Mechanical Analogy
3.5 Generalization to Other Energy Systems
3.6 Summary and Discussions
Chapter 4 Synchronverter Based Generation
4.1 Mathematical Model of Synchronous Generatorss
4.1.1 The Electrical Part
4.1.2 The Mechanical Part
4.1.3 Presence of a Neutral Line
4.2 Implementation of a Synchronverter
4.2.1 The Power Part
4.2.2 The Electronic Part
4.3 Operation of a Synchronverter. 4.3.1 Regulation of Real Power and Frequency Droop Control
4.3.2 Regulation of Reactive Power and Voltage Droop Control
4.4 Simulation Results
4.4.1 Under Different Grid Frequencies
4.4.2 Under Different Load Conditions
4.5 Experimental Results
4.5.1 Grid‐connected Set Mode
4.5.2 Grid‐connected Droop Mode
4.5.3 Grid‐connected Parallel Operation
4.5.4 Seamless Transfer of the Operation Mode
4.6 Summary
Chapter 5 Synchronverter Based Loads
5.1 Introduction
5.2 Modeling of a Synchronous Motor
5.3 Operation of a PWM Rectifier as a VSM
5.3.1 Controlling the Power
5.3.2 Controlling the DC‐bus Voltage
5.4 Simulation Results
5.4.1 Controlling the Power
5.4.2 Controlling the DC‐bus Voltage
5.5 Experimental Results
5.5.1 Controlling the Power
5.5.2 Controlling the DC‐bus Voltage
5.6 Summary
Chapter 6 Control of Permanent Magnet Synchronous Generator (PMSG) Based Wind Turbines
6.1 Introduction
6.2 PMSG Based Wind Turbines
6.3 Control of the Rotor‐Side Converter
6.4 Control of the Grid‐Side Converter
6.5 Real‐time Simulation Results
6.5.1 Under Normal Grid Conditions
6.5.1.1 GSC Performance
6.5.1.2 RSC Performance
6.5.2 Under Grid Faults
6.6 Summary
Chapter 7 Synchronverter Based AC Ward Leonard Drive Systems
7.1 Introduction
7.2 Ward Leonard Drive Systems
7.3 Model of a Synchronous Generator
7.4 Control Scheme with a Speed Sensor. 7.4.1 Control Structure
7.4.2 System Analysis and Parameter Selection
7.5 Control Scheme without a Speed Sensor. 7.5.1 Control Structure
7.5.2 System Analysis and Parameter Selection
7.6 Experimental Results
7.6.1 Case 1: With a Speed Sensor for Feedback
7.6.1.1 Reversal from a High Speed without a Load
7.6.1.2 Reversal from a High Speed with a Load
7.6.1.3 Reversal from a Low Speed without a Load
7.6.1.4 Reversal from a Low Speed with a Load
7.6.1.5 Reversal at an Extremely Low Speed without a Load
7.6.2 Case 2: Without a Speed Sensor for Feedback
7.6.2.1 Reversal from a High Speed without a Load
7.6.2.2 Reversal from a High Speed with a Load
7.7 Summary
Note
Chapter 8 Synchronverter without a Dedicated Synchronization Unit
8.1 Introduction
8.2 Interaction of a Synchronous Generator (SG) with an Infinite Bus
8.3 Controller for a Self‐synchronized Synchronverter
8.3.1 Operation after Connection to the Grid
8.3.2 Synchronization before Connection to the Grid
8.4 Simulation Results
8.4.1 Normal Operation
8.4.2 Operation under Grid Faults
8.5 Experimental Results
8.5.1 Case 1: With the Grid Frequency Below 50 Hz
8.5.2 Case 2: With the Grid Frequency Above 50 Hz
8.6 Benefits of Removing the Synchronization Unit
8.7 Summary
Notes
Chapter 9 Synchronverter Based Loads without a Dedicated Synchronisation Unit
9.1 Controlling the DC‐bus Voltage
9.1.1 Self‐synchronization
9.1.2 Normal Operation
9.2 Controlling the Power
9.3 Simulation Results
9.3.1 Controlling the DC‐bus Voltage
9.3.2 Controlling the Power
9.4 Experimental Results
9.4.1 Controlling the DC‐bus Voltage
9.4.2 Controlling the Power
9.5 Summary
Chapter 10 Control of a DFIG Based Wind Turbine as a VSG (DFIG‐VSG)
10.1 Introduction
10.2 DFIG Based Wind Turbines
10.3 Differential Gears and Ancient Chinese South‐pointing Chariots
10.4 Analogy between a DFIG and Differential Gears
10.5 Control of a Grid‐side Converter
10.5.1 DC‐bus Voltage Control
10.5.2 Unity Power Factor Control
10.5.3 Self‐synchronization
10.6 Control of the Rotor‐Side Converter
10.6.1 Frequency Control
10.6.2 Voltage Control
10.6.3 Self‐synchronization
10.7 Regulation of System Frequency and Voltage
10.8 Simulation Results
10.9 Experimental Results
10.10 Summary
Chapter 11 Synchronverter Based Transformerless Photovoltaic Systems
11.1 Introduction
11.2 Leakage Currents and Grounding of Grid‐tied Converters. 11.2.1 Ground, Grounding, and Grounded Systems
11.2.2 Leakage Currents in a Grid‐tied Converter
11.2.3 Benefits of Providing a Common AC and DC Ground
11.3 Operation of a Conventional Half‐bridge Inverter
11.3.1 Reduction of Leakage Currents
11.3.2 Output Voltage Range
11.4 A Transformerless PV Inverter. 11.4.1 Topology
11.4.2 Control of the Neutral Leg
11.4.3 Control of the Inversion Leg as a VSM
11.4.3.1 Real Power Control and Frequency Droop
11.4.3.2 Reactive Power Control and Voltage Droop
11.4.3.3 Synchronization
11.5 Real‐time Simulation Results
11.6 Summary
Chapter 12 Synchronverter Based STATCOM without an Dedicated Synchronization Unit
12.1 Introduction
12.2 Conventional Control of STATCOM
12.2.1 Operational Principles
12.2.2 Typical Control Strategy
12.3 Synchronverter Based Control
12.3.1 Regulation of the DC‐bus Voltage and Synchronization with the Grid
12.3.2 Operation in the ‐mode to Regulate the Reactive Power
12.3.3 Operation in the ‐mode to Regulate the PCC Voltage
12.3.4 Operation in the ‐mode to Droop the Voltage
12.4 Simulation Results. 12.4.1 System Description
12.4.2 Connection to the Grid
12.4.3 Normal Operation in Different Modes
12.4.4 Operation under Extreme Conditions. 12.4.4.1 With a Changing Grid Frequency
12.4.4.2 With a Changing Grid Voltage
12.4.4.3 With a Changing System Strength
12.5 Summary
Chapter 13 Synchronverters with Bounded Frequency and Voltage
13.1 Introduction
13.2 Model of the Original Synchronverter
13.3 Achieving Bounded Frequency and Voltage
13.3.1 Control Design
13.3.1.1 Maintaining the Frequency within a Given Range
13.3.1.2 Maintaining the Voltage within a Given Range
13.3.2 Existence of a Unique Equilibrium
13.3.2.1 Theoretical Analysis
13.3.2.2 A Numerical Example
13.3.3 Convergence to the Equilibrium
13.3.3.1 Stability of the Fast Dynamics
13.3.3.2 Stability of the Slow Dynamics
13.4 Real‐time Simulation Results
13.5 Summary
Chapter 14 Virtual Inertia, Virtual Damping, and Fault Ride‐through
14.1 Introduction
14.2 Inertia, the Inertia Time Constant, and the Inertia Constant
14.3 Limitation of the Inertia of a Synchronverter
14.4 Reconfiguration of the Inertia Time Constant. 14.4.1 Design and Outcome
14.4.2 What is the Catch?
14.5 Reconfiguration of the Virtual Damping
14.5.1 Through Impedance Scaling with an Inner‐loop Voltage Controller. 14.5.1.1 Controller Design and Analysis
14.5.1.2 Enhancement of Voltage Quality
14.5.2 Through Impedance Insertion with an Inner‐loop Current Controller
14.6 Fault Ride‐through. 14.6.1 Analysis
14.6.2 Recommended Design
14.7 Simulation Results
14.7.1 A Single VSM
14.7.1.1 Reconfigurability of Virtual Inertia and Virtual Damping
14.7.1.2 Effect of the Virtual Damping
14.7.2 Two VSMs in Parallel Operation
14.7.2.1 Case 1: s
14.7.2.2 Case 2:
14.7.2.3 Fault Ride‐through
14.8 Experimental Results
14.8.1 A Single VSM
14.8.1.1 Reconfigurability of the Inertia Time Constant and Damping
14.8.1.2 Effect of the Virtual Damping
14.8.2 Two VSMs in Parallel Operation
14.9 Summary
Chapter 15 Synchronization Mechanism of Droop Control
15.1 Brief Review of Phase‐Locked Loops (PLLs) 15.1.1 Basic PLL
15.1.2 Enhanced PLL (EPLL)
15.2 Brief Review of Droop Control
15.3 Structural Resemblance between Droop Control and PLL. 15.3.1 When the Impedance is Inductive
15.3.2 When the Impedance is Resistive
15.4 Operation of a Droop Controller as a Synchronization Unit
15.5 Experimental Results
15.5.1 Synchronization with the Grid
15.5.2 Connection to the Grid
15.5.3 Operation in the Droop Mode
15.5.4 Robustness of Synchronization
15.5.5 Change in the Operation Mode
15.6 Summary
Note
16 Robust Droop Control
16.1 Control of Inverter Output Impedance
16.1.1 Inverters with Inductive Output Impedances (L‐inverters)
16.1.2 Inverters with Resistive Output Impedances (R‐inverters)
16.1.3 Inverters with Capacitive Output Impedances (C‐inverters)
16.2 Inherent Limitations of Conventional Droop Control. 16.2.1 Basic Principle
16.2.2 Experimental Phenomena
16.2.3 Real Power Sharing
16.2.4 Reactive Power Sharing
16.3 Robust Droop Control of R‐inverters. 16.3.1 Control Strategy
16.3.2 Error due to Inaccurate Voltage Measurements
16.3.3 Voltage Regulation
16.3.4 Error due to the Global Settings for and
16.3.5 Experimental Results
16.3.5.1 Inverters having Different Per‐unit Output Impedances with a Linear Load
16.3.5.2 Inverters having the Same Per‐unit Output Impedance with a Linear Load
16.3.5.3 With a Nonlinear Load
16.4 Robust Droop Control of C‐inverters. 16.4.1 Control Strategy
16.4.2 Experimental Results
16.4.2.1 With a Linear Load
16.4.2.2 With a Nonlinear Load
16.5 Robust Droop Control of L‐inverters. 16.5.1 Control Strategy
16.5.2 Experimental Results
16.5.2.1 With a Linear Load
16.5.2.2 With a Nonlinear Load
16.6 Summary
17 Universal Droop Control
17.1 Introduction
17.2 Further Insights into Droop Control
17.2.1 Parallel Operation of Inverters with the Same Type of Impedance
17.2.2 Parallel Operation of L‐, R‐, and ‐inverters
17.2.3 Parallel Operation of ‐, R‐, and C‐inverters
17.3 Universal Droop Controller. 17.3.1 Basic Principle
17.3.2 Implementation
17.4 Real‐time Simulation Results
17.5 Experimental Results
17.5.1 Case I: Parallel Operation of L‐ and C‐inverters
17.5.2 Case II: Parallel Operation of L‐, C‐, and R‐inverters
17.6 Summary
18 Self‐synchronized Universal Droop Controller
18.1 Description of the Controller
18.2 Operation of the Controller. 18.2.1 Self‐synchronization Mode
18.2.2 Set Mode (‐mode and ‐mode)
18.2.3 Droop Mode (‐mode and ‐mode)
18.3 Experimental Results
18.3.1 R‐inverter with Self‐synchronized Universal Droop Control. 18.3.1.1 Self‐synchronization
18.3.1.2 Connection to the Grid
18.3.1.3 Regulation of Real and Reactive Power
18.3.1.4 Impact of Change in the DC‐bus Voltage
18.3.2 L‐inverter with Self‐synchronized Universal Droop Control. 18.3.2.1 Self‐synchronization
18.3.2.2 Connection to the Grid
18.3.2.3 Regulation of Real and Reactive Power
18.3.2.4 Impact of the Change in the DC‐bus Voltage
18.3.3 L‐inverter with Self‐synchronized Robust Droop Control
18.3.3.1 Self‐synchronization
18.3.3.2 Connection to the Grid
18.3.3.3 Regulation of Real and Reactive Power
18.3.3.4 Impact of the Change in the DC‐bus Voltage
18.4 Real‐time Simulation Results from a Microgrid
18.5 Summary
19 Droop‐Controlled Loads for Continuous Demand Response
19.1 Introduction
19.2 Control Framework with a Three‐port Converter. 19.2.1 Generation of the Real Power Reference
19.2.2 Regulation of the Power Drawn from the Grid
19.2.3 Analysis of the Operation Modes
19.2.4 Determination of the Capacitance for Grid Support
19.3 An Illustrative Implementation with the ‐converter
19.3.1 Brief Description about the ‐converter
19.3.2 Control of the Neutral Leg
19.3.3 Control of the Conversion Leg
19.4 Experimental Results
19.4.1 Design of the Experimental System
19.4.2 Steady‐state Performance. 19.4.2.1 Operation under the Grid‐support Mode
19.4.2.2 Operation under the No‐support Mode
19.4.3 Transient Performance. 19.4.3.1 System Start‐up
19.4.3.2 Connection of the Load
19.4.4 Capacity Potential
19.4.5 Comparative Study
19.5 Summary
20 Current‐limiting Universal Droop Controller
20.1 Introduction
20.2 System Modeling
20.3 Control Design. 20.3.1 Structure
20.3.2 Implementation
20.4 System Analysis
20.4.1 Current‐limiting Property
20.4.2 Closed‐loop Stability
20.4.3 Selection of Control Parameters
20.5 Practical Implementation
20.6 Operation under Grid Variations and Faults
20.7 Experimental Results
20.7.1 Operation under Normal Conditions
20.7.2 Operation under Grid Faults
20.8 Summary
21 Cybersync Machines
21.1 Introduction
21.2 Passivity and Port‐Hamiltonian Systems. 21.2.1 Passive Systems
21.2.2 Port‐Hamiltonian Systems
21.2.3 Passivity of Interconnected Passive Systems
21.3 System Modeling
21.4 Control Framework
21.4.1 The Engendering Block
21.4.2 Generation of the Desired Frequency and Flux
21.4.3 Design of and to Obtain a Passive
21.5 Passivity of the Controller
21.5.1 Losslessness of the Interconnection Block
21.5.2 Passivity of the Cascade of and
21.6 Passivity of the Closed‐loop System
21.7 Sample Implementations for Blocks and
21.7.1 Using the Standard Integral Controller (IC)
21.7.2 Using a Static Controller
21.8 Self‐Synchronization and Power Regulation
21.9 Simulation Results
21.9.1 Self‐synchronization
21.9.2 Operation after Connection to the Grid
21.10 Experimental Results
21.10.1 Self‐synchronization
21.10.2 Operation after Connection to the Grid
21.11 Summary
22 A Single‐node System
22.1 SYNDEM Smart Grid Research and Educational Kit. 22.1.1 Overview
22.1.2 Hardware Structure
22.1.2.1 Power Board
22.1.2.2 Control Board
22.1.3 Sample Conversion Topologies Attainable
22.1.3.1 DC–DC Converters
22.1.3.2 Uncontrolled Rectifiers
22.1.3.3 PWM‐controlled Rectifiers
22.1.3.4 ‐Converters
22.1.3.5 Inverters
22.1.3.6 DC–DC–AC Converters
22.1.3.7 Single‐phase AC–DC–AC Back‐to‐Back Converters
22.1.3.8 Three‐phase AC–DC–AC Back‐to‐Back Converters
22.2 Details of the Single‐Node SYNDEM System. 22.2.1 Description of the System
22.2.2 Experimental Results
22.3 Summary
Note
23 A 100% Power Electronics Based SYNDEM Smart Grid Testbed
23.1 Description of the Testbed. 23.1.1 Overall Structure
23.1.2 VSM Topologies Adopted
23.1.2.1 ‐Converter
23.1.2.2 Beijing Converter
23.1.3 Individual Nodes. 23.1.3.1 Energy Bridges
23.1.3.2 Solar Power Nodes
23.1.3.3 Wind Power Nodes
23.1.3.4 DC‐Load Node
23.1.3.5 AC‐Load Node
23.2 Experimental Results
23.2.1 Operation of Energy Bridges
23.2.2 Operation of Solar Power Nodes
23.2.3 Operation of Wind Power Nodes
23.2.4 Operation of the DC‐Load Node
23.2.5 Operation of the AC‐Load Node
23.2.6 Operation of the Whole Testbed
23.3 Summary
24 A Home Grid
24.1 Description of the Home Grid
24.2 Results from Field Operations
24.2.1 Black start and Grid forming
24.2.2 From Islanded to Grid‐tied Operation
24.2.3 Seamless Mode Change when the Public Grid is Lost and Recovered
24.2.4 Voltage/Frequency Regulation and Power Sharing
24.3 Unexpected Problems Emerged During the Field Trial
24.4 Summary
25 Texas Panhandle Wind Power System
25.1 Geographical Description
25.2 System Structure
25.3 Main Challenges
25.4 Overview of Control Strategies Compared
25.4.1 VSM Control
25.4.2 DQ Control
25.5 Simulation Results
25.5.1 VSM Control
25.5.2 DQ Control
25.6 Summary and Conclusions
Bibliography
Index
WILEY END USER LICENSE AGREEMENT
Отрывок из книги
Next Generation Smart Grids
Qing-Chang Zhong Illinois Institute of Technology & Syndem LLC Chicago, USA
.....
Figure 15.2 Enhanced phase‐locked loop (EPLL) or sinusoidal tracking algorithm (STA).
Figure 15.3 Power delivery to a voltage source through an impedance.
.....