Smart Solar PV Inverters with Advanced Grid Support Functionalities
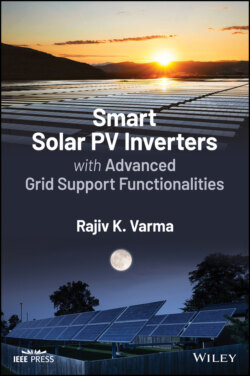
Реклама. ООО «ЛитРес», ИНН: 7719571260.
Оглавление
Rajiv K. Varma. Smart Solar PV Inverters with Advanced Grid Support Functionalities
Table of Contents
List of Tables
List of Illustrations
Guide
Pages
SMART SOLAR PV INVERTERS. WITH ADVANCED GRID SUPPORT FUNCTIONALITIES
ABOUT THE AUTHOR
FOREWORD
PREFACE
ACKNOWLEDGMENTS
LIST OF ABBREVIATIONS
1 IMPACTS OF HIGH PENETRATION OF SOLAR PV SYSTEMS AND SMART INVERTER DEVELOPMENTS
1.1 Concepts of Reactive and Active Power Control. 1.1.1 Reactive Power Control. 1.1.1.1 Voltage Control
1.1.1.2 Frequency Control
1.1.2 Active Power Control. 1.1.2.1 Voltage Control
1.1.2.2 Frequency Control
1.1.3 Frequency Response with Synchronous Machines
1.1.3.1 Rate of Change of Frequency
1.1.3.2 Factors Impacting ROCOF
1.1.3.3 System Inertia
1.1.3.4 Critical Inertia
1.1.3.5 Size of Largest Contingency
1.1.4 Fast Frequency Response
1.2 Challenges of High Penetration of Solar PV Systems
1.2.1 Steady‐state Overvoltage
1.2.2 Voltage Fluctuations
1.2.3 Reverse Power Flow
1.2.4 Transient Overvoltage
1.2.5 Voltage Unbalance
1.2.6 Decrease in Voltage Support Capability of Power Systems
1.2.7 Interaction with Conventional Voltage Regulation Equipment
1.2.8 Variability of Power Output
1.2.9 Balancing Supply and Demand
1.2.10 Changes in Active Power Flow in Feeders
1.2.11 Change in Reactive Power Flow in Feeders
1.2.12 Line Losses
1.2.13 Harmonic Injections
1.2.14 Low Short Circuit Levels
1.2.15 Protection and Control Issues
1.2.16 Short Circuit Current Issues
1.2.17 Unintentional Islanding
1.2.18 Frequency Regulation Issues due to Reduced Inertia
1.2.18.1 Under Frequency Response
1.2.18.2 Over Frequency Response
1.2.19 Angular Stability Issues due to Reduced Inertia
1.3 Development of Smart Inverters
1.3.1 Developments in Germany
1.3.2 Developments in the USA
1.3.3 Development in Canada of Night and Day Control of Solar PV Farms as STATCOM (PV‐STATCOM)
1.4 Conclusions
References
2 SMART INVERTER FUNCTIONS
2.1 Capability Characteristics of Distributed Energy Resource (DER)
Reactive Power Capability Characteristic of a Synchronous Generator
2.2 General Considerations in Implementation of Smart Inverter Functions
2.2.1 Performance Categories
2.2.1.1 Normal Performance
2.2.1.2 Abnormal Performance
2.2.2 Reactive Power Capability of DERs
2.2.2.1 Active Power (Watt) Precedence Mode
2.2.2.2 Reactive Power (Var) Precedence Mode
2.3 Smart Inverter Functions for Reactive Power and Voltage Control. 2.3.1 Constant Power Factor Function
2.3.2 Constant Reactive Power Function
2.3.3 Voltage–Reactive Power (Volt–Var) Function
2.3.4 Active Power–Reactive Power (Watt–Var or P–Q) Function
2.3.5 Dynamic Voltage Support Function
2.3.5.1 Dynamic Network Support Function
2.3.5.2 Dynamic Reactive Current Support Function
2.4 Smart Inverter Function for Voltage and Active Power Control. 2.4.1 Voltage–Active Power (Volt–Watt) Function
2.4.2 Coordination with Volt–Var Function
2.4.3 Dynamic Volt–Watt Function
2.5 Low/High Voltage Ride‐Through (L/H VRT) Function
2.5.1 IEEE Standard 1547‐2018
2.5.2 North American Electric Reliability Corporation (NERC) Standard PRC‐024
2.6 Frequency–Watt Function
2.6.1 Frequency–Watt Function 1
2.6.2 Frequency–Watt Function 2
2.6.3 Frequency Droop Function
2.6.4 Frequency–Watt Function with Energy Storage
2.7 Low/High Frequency Ride‐Through (L/H FRT) Function
2.7.1 IEEE Standard 1547‐2018
2.7.2 North American Electric Reliability Corporation (NERC) Standard PRC‐024
2.8 Ramp Rate
2.9 Fast Frequency Response
2.10 Smart Inverter Functions Related to DERs Based on Energy Storage Systems. 2.10.1 Direct Charge/Discharge Function
2.10.2 Price‐Based Charge/Discharge Function
2.10.3 Coordinated Charge/Discharge Management Function
2.10.3.1 Time‐Based Charging Model
2.10.3.2 Duration at Maximum Charging and Discharging Rates
2.11 Limit Maximum Active Power Function. 2.11.1 Without Energy Storage
2.11.2 With Energy Storage System
2.12 Set Active Power Mode
2.13 Active Power Smoothing Mode
2.14 Active Power Following Function
2.15 Prioritization of Different Functions
2.15.1 Active Power‐related Functions
2.15.1.1 Functions Affecting Operating Boundaries
2.15.1.2 Dynamic Functions
2.15.1.3 Steady‐State Functions Managing Watt Input/Output
2.15.2 Reactive Power‐Related Functions
2.15.2.1 Dynamic Functions
2.15.2.2 Steady‐State Functions
2.15.3 Smart Inverter Functions Under Abnormal Conditions
2.16 Emerging Functions
2.16.1 PV‐STATCOM: Control of PV inverters as STATCOM during Night and Day
2.16.2 Reactive Power at No Active Power Output
2.17 Summary
References
3 MODELING AND CONTROL OF THREE‐PHASE SMART PV INVERTERS
3.1 Power Flow from a Smart Inverter System
3.1.1 Active Power Flow. 3.1.1.1 Magnitude of Active Power Flow
3.1.1.2 Direction of Active Power Flow
3.1.2 Reactive Power Flow
3.1.2.1 Magnitude of Reactive Power Flow
3.1.2.2 Direction of Reactive Power Flow
3.1.3 Implementation of Smart Inverter Functions
3.2 Smart PV Inverter System
3.3 Power Circuit Constituents of Smart Inverter System
3.3.1 PV Panels
3.3.2 Maximum Power Point Tracking (MPPT) Scheme
3.3.3 Non‐MPP Voltage Control
3.3.4 Voltage Source Converter (VSC)
3.3.4.1 DC‐Link Capacitor
3.3.4.2 AC Filter
3.3.4.3 Isolation Transformer
3.4 Control Circuit Constituents of Smart Inverter System
3.4.1 Measurement Filters
3.4.2 abc‐dq Transformation. 3.4.2.1 Concept
3.4.2.2 Theoretical Basis
3.4.2.3 Power in abc and dq Reference Frame
3.4.3 Pulse Width Modulation (PWM)
3.4.4 Phase Locked Loop (PLL)
Effect of PLL on Active and Reactive Power Output of VSC
3.4.5 Current Controller
3.4.6 DC‐Link Voltage Controller
3.5 Smart Inverter Voltage Controllers
3.5.1 Volt–Var Control
3.5.2 Closed‐Loop Voltage Controller
3.6 PV Plant Control
3.7 Modeling Guidelines
3.8 Summary
References
4 PV‐STATCOM: A NEW SMART PV INVERTER AND A NEW FACTS CONTRO
4.1 Flexible AC Transmission System (FACTS)
4.2 Static Var Compensator (SVC)
Control System of SVC
4.3 Synchronous Condenser
4.4 Static Synchronous Compensator (STATCOM)
Control System of STATCOM
4.5 Control Modes of SVC and STATCOM
4.5.1 Dynamic Voltage Regulation
4.5.1.1 Power Transfer Without Midpoint Voltage Regulation
4.5.1.2 Power Transfer with Midpoint Voltage Regulation
4.5.2 Modulation of Bus Voltage in Response to System Oscillations
Damping of Power Oscillations with Reactive Power Control
4.5.3 Load Compensation
4.6 Photovoltaic‐Static Synchronous Compensator (PV‐STATCOM)
4.7 Operating Modes of PV‐STATCOM
4.7.1 Nighttime
4.7.2 Daytime with Active Power Priority
4.7.3 Daytime with Reactive Power Priority
4.7.3.1 Reactive Power Modulation After Full Active Power Curtailment
4.7.3.2 Reactive Power Modulation After Partial Active Power Curtailment
4.7.3.3 Simultaneous Active and Reactive Power Modulation After Partial Active Power Curtailment
4.7.3.4 Simultaneous Active and Reactive Power Modulation with Pre‐existing Active Power Curtailment
4.7.4 Methodology of Modulation of Active Power
4.8 Functions of PV‐STATCOM. 4.8.1 A New Smart Inverter
4.8.2 A New FACTS Controller
4.9 Cost of Transforming an Existing Solar PV System into PV‐STATCOM
4.9.1 Constituents of a PV System
4.9.2 Costing of PV‐STATCOM
4.9.2.1 Cost of 5 Mvar PV‐STATCOM
4.9.2.2 Cost of 100 Mvar PV‐STATCOM
4.9.3 Cost of a STATCOM
4.9.3.1 Equipment Cost
4.9.3.2 Infrastructure Costs
4.10 Cost of Operating a PV‐STATCOM. 4.10.1 Nighttime Operating Costs
4.10.2 Daytime Operating Costs
4.10.3 Additional Costs
4.10.4 Technical Considerations of PV‐STATCOM and STATCOM
4.10.4.1 Number of Inverters
4.10.4.2 Ability to Provide Full Reactive Power at Nighttime
4.10.4.3 Transient Overvoltage and Overcurrent Rating
4.10.4.4 Low Voltage Ride‐through
4.10.5 Potential of PV‐STATCOM
4.11 Summary
References
5 PV‐STATCOM APPLICATIONS IN DISTRIBUTION SYSTEMS
5.1 Nighttime Application of PV Solar Farm as STATCOM to Regulate Grid Voltage
5.1.1 Modeling of Solar PV System
5.1.2 Solar Farm Inverter Control
5.1.3 Simulation Study
5.1.4 Summary
5.2 Increasing Wind Farm Connectivity with PV‐STATCOM
5.2.1 Study System
5.2.2 Control System
5.2.3 Model of Wind Farm
5.2.4 Simulation Studies
5.2.4.1 Mitigation of Steady‐state Voltage Rise
5.2.4.2 Control of Transient Overvoltage
5.2.4.3 PV‐STATCOM Reactive Power Requirement
5.2.4.4 Effect of Distance of PV‐STATCOM from Wind Farm
5.2.4.5 Increase in Wind Farm Connectivity
5.2.5 Summary
5.3 Dynamic Voltage Control by PV‐STATCOM
5.3.1 Study System
5.3.2 Control System
5.3.2.1 DC‐link Voltage Control
5.3.2.2 AC Voltage Control
5.3.2.3 Power Factor Control (PFC)
5.3.3 Operation Mode Selector
5.3.4 PSCAD Simulation Studies
5.3.4.1 Full STATCOM Mode – Daytime
5.3.4.2 Full STATCOM Mode – Nighttime
5.3.4.3 Low‐voltage Ride‐through (LVRT)
5.3.5 Summary
5.4 Enhancement of Solar Farm Connectivity by PV‐STATCOM
5.4.1 Study System
5.4.2 System Modeling
5.4.3 Control System
5.4.3.1 Operation Mode Selector
5.4.3.2 PCC Voltage Control
5.4.3.3 TOV Detection Block
5.4.4 Simulation Studies
5.4.4.1 Conventional PV System (Without PV‐STATCOM Control)
5.4.4.2 PV‐STATCOM and Two Conventional Solar PV Systems
(a) Single Line‐to‐Ground (SLG) Fault
(b) Line‐to‐Line‐to‐Ground (LLG) Fault
5.4.5 Summary
5.5 Reduction of Line Losses by PV‐STATCOM
5.5.1 Concept of PV‐STATCOM Voltage Control for Line Loss Reduction
5.5.1.1 Determination of Optimal Voltage Setpoints
5.5.1.2 Inverter Operating Losses
5.5.2 Simulation Studies
5.5.2.1 Case Study 1: Two Bus Radial System with Constant Load
5.5.2.2 Case Study II: IEEE 33 Bus System with Variable Load
5.5.2.3 Improvement in Loss Reduction with PV‐STATCOM
5.5.3 Summary
5.6 Stabilization of a Remotely Located Critical Motor by PV‐STATCOM
5.6.1 Study Systems. 5.6.1.1 Study System 1
5.6.1.2 Study System 2
5.6.2 Study System with PV‐STATCOM Control. 5.6.2.1 Grid‐Connected Solar PV Plant
5.6.2.2 Conventional PV Inverter Control
5.6.2.3 PV‐STATCOM Controller
Operation Mode Selector
Active Power Controller
Reactive Power Controller
Nighttime Voltage Controller
5.6.3 Simulation Studies on Study System 1
5.6.3.1 Performance of the Proposed PV‐STATCOM Controller
5.6.3.2 Comparison of PV‐STATCOM and STATCOM Operation
5.6.4 Field Validation Tests on Utility Solar PV System
5.6.4.1 PV Solar Plant Without PV‐STATCOM Control
5.6.4.2 PV Solar System Operation According to German Grid Code
5.6.4.3 PV Solar System Operating as PV‐STATCOM at Night
5.6.5 Simulation Studies on Study System 2
5.6.6 Summary
5.7 Conclusions
References
6 PV‐STATCOM APPLICATIONS IN TRANSMISSION SYSTEMS
6.1 Increasing Power Transmission Capacity by PV‐STATCOM
6.1.1 Study Systems
6.1.2 System Model
6.1.3 Control System. 6.1.3.1 Conventional Reactive Power Control
6.1.3.2 PCC Voltage Control
6.1.3.3 Damping Control
6.1.4 Power Transfer Studies for Study System I
6.1.4.1 Nighttime Operation of Solar PV System as PV‐STATCOM
6.1.4.2 Daytime Operation of Solar PV System as PV‐STATCOM
6.1.5 Power Transfer Studies for Study System II
6.1.5.1 Nighttime Operation of Solar DG and Wind DG as STATCOM
6.1.5.2 Daytime Operation of Solar DG and Wind DG as STATCOM
6.1.6 Summary
6.2 Power Oscillation Damping by PV‐STATCOM
6.2.1 Study System
6.2.2 PV‐STATCOM Control System
6.2.2.1 DC Voltage Controller
6.2.2.2 Conventional PV Controller
6.2.2.3 Q‐POD Controller
6.2.2.4 Oscillation Detection Unit (ODU)
6.2.2.5 PV Active Power Controllers
Power Restoration in a Ramped Manner
Power Restoration in the Partial STATCOM Mode with POD Control Active
6.2.2.6 Design of POD Controller
6.2.2.7 Small Signal Studies of the POD Control
6.2.3 Simulations Studies. 6.2.3.1 Power Transfer without PV‐STATCOM Control
6.2.3.2 Power Transfer with Full STATCOM Damping Control and Power Restoration in Normal Ramped Manner
6.2.3.3 Power Transfer with Full STATCOM Damping Control and Ramped Power Restoration with POD Control Active in Partial STATCOM Mode
6.2.3.4 Nighttime Power Transfer Enhancement with Full STATCOM POD Control
6.2.3.5 Effect of PV‐STATCOM Control on System Frequency
6.2.4 Summary
6.3 Power Oscillation Damping with Combined Active and Reactive Power Modulation Control of PV‐STATCOM
6.3.1 Modes of PV‐STATCOM Control
6.3.1.1 Partial STATCOM
6.3.1.2 Full STATCOM
6.3.2 Study System
6.3.3 PV‐STATCOM Control System
6.3.4 PV Reactive Power Controllers. 6.3.4.1 Conventional Reactive Power Control
6.3.4.2 Q‐POD Controller
6.3.5 PV‐Active Power Controllers. 6.3.5.1 Conventional Active Power Control
6.3.5.2 P‐POD Controller
6.3.5.3 PQ‐POD Controller
6.3.5.4 Active Power Restoration Controller
6.3.6 Small Signal PV‐STATCOM Modeling
6.3.7 Selection of PV‐STATCOM Controller Mode
6.3.8 Design of POD Controllers
6.3.9 Effect of PV‐STATCOM Placement on Effectiveness of POD Techniques
6.3.9.1 Residue Analysis for PV‐STATCOM with Q‐POD
6.3.9.2 Residue Analysis for PV‐STATCOM with P‐POD
6.3.10 Simulation Studies
6.3.10.1 POD by PV‐STATCOM
6.3.10.2 Effect of POD Controllers on System Frequency
6.3.10.3 Effect of PV Active Power Output on POD Controls
6.3.10.4 POD by PV‐STATCOM Connected at Other Buses
6.3.11 Summary
6.4 Mitigation of Subsynchronous Resonance (SSR) in Synchronous Generator by PV‐STATCOM
6.4.1 Study System
6.4.2 Control System
6.4.3 SSR Damping Controller
6.4.4 DC Voltage Controller
6.4.5 Simulation Studies
6.4.5.1 Damping of Critical Mode 1 (67% Series Compensation)
6.4.5.2 Ramp Up without PV‐STATCOM Control
6.4.5.3 Damping of Critical Mode 2 (54% Series Compensation)
6.4.5.4 Damping of Critical Mode 3 (41% Series Compensation)
6.4.5.5 Damping of Critical Mode 4 (26% Series Compensation)
6.4.6 Potential of Utilizing Large Solar Farms for Damping SSR
6.4.7 Summary
6.5 Alleviation of Subsynchronous Oscillations (SSOs) in Induction‐Generator‐Based Wind Farm by PV‐STATCOM
6.5.1 Study System
6.5.2 Control System. 6.5.2.1 Current Controller
6.5.2.2 Damping Controller
6.5.2.3 DC Voltage Controller
6.5.3 Simulation Studies. 6.5.3.1 Daytime Case Study: PV System Connected at Wind Farm Terminal
6.5.3.2 Daytime Case Study: PV System Connected at Line Midpoint
6.5.3.3 Nighttime Case Study: SSO Alleviation by PV‐STATCOM
6.5.4 Potential of Utilizing Large Solar Farms for Alleviating SSO in Wind Farms
6.5.5 Summary
6.6 Mitigation of Fault‐Induced Delayed Voltage Recovery (FIDVR) by PV‐STATCOM
6.6.1 Study System
6.6.2 Structure of a Large Utility‐Scale Solar PV Plant
6.6.3 Proposed PV‐STATCOM Control
6.6.3.1 Mode Selector
6.6.3.2 Sensitivity Calculator
6.6.3.3 Current Reference Calculator
6.6.4 Design of PV‐STATCOM Controllers
6.6.5 Simulation Studies
6.6.5.1 Response of IMs for LLL‐G Fault with no PV Plant Control
6.6.5.2 Performance of Proposed PV‐STATCOM Controller
6.6.5.3 Advantage of Enhanced Voltage Support up to TOV Limit
6.6.5.4 Comparison of Proposed PV STATCOM Controller and Other Smart Inverter Controls
6.6.5.5 Comparison of PV STATCOM Controller and STATCOM
6.6.5.6 PV‐STATCOM Impact on System Frequency
6.6.5.7 Nighttime Performance of PV‐STATCOM Controller
6.6.5.8 Compliance with IEEE Standard 1547–2018
6.6.6 Summary
6.7 Simultaneous Fast Frequency Control and Power Oscillation Damping by PV‐STATCOM
6.7.1 Study System
6.7.2 System Modeling. 6.7.2.1 PV Plant Model
6.7.2.2 Combined FFR and POD Controller
Modified Plant Controller (Repc)
Plant Power Limit Logic
Power Oscillation Damping (POD) Controller
Fast Frequency Response (FFR) Controller
6.7.3 Simulation Scenarios
6.7.4 Over‐Frequency Control
6.7.4.1 25 MW Load Trip in Area 1 (Pavailable = 100 MW, Kcurt = 0); (Power Imbalance less than PV Plant Capacity)
6.7.4.2 200 MW Load Trip in Area 1 (Pavailable = 100 MW, Kcurt = 0); (Power Imbalance more than PV Plant Capacity)
6.7.5 Under Frequency Control
6.7.5.1 25 MW Load Connection in Area 1 (Pavailable = 100 MW, Kcurt = 50%); (Power Imbalance less than PV Plant Capacity)
6.7.5.2 100 MW Load Connection in Area 1(Pavailable = 100 MW, Kcurt = 50%); (Power Imbalance more than PV Plant Capacity)
6.7.6 Performance Comparison of Proposed FFR + POD Control with Conventional Frequency Control
6.7.7 Summary
6.8 Conclusions
References
7 INCREASING HOSTING CAPACITY BY SMART INVERTERS – CONCEPTS AND APPLICATIONS
7.1 Hosting Capacity of Distribution Feeders
7.1.1 Voltage
7.1.2 Thermal Overloading
7.2 Hosting Capacity Based on Voltage Violations
Variants of Hosting Capacity
7.3 Increasing Hosting Capacity with Non Smart Inverter Techniques
7.3.1 Active Power Curtailment (APC)
7.3.2 Change in Orientation of PV Panels
7.3.3 Correlation between Load and PV Systems
7.3.4 Demand Side Management (DSM)
7.3.5 On Load Tap Changer (OLTC) Transformers, Voltage Regulators, and Switched Capacitors
7.3.6 Application of Decentralized Energy Storage Systems
7.3.7 Energy Storage Requirements for Achieving 50% Solar PV Energy Penetration in California
7.3.8 Comparative Evaluation of Different Techniques for Increasing Hosting Capacity
7.3.8.1 Active Power Curtailment
7.3.8.2 Different PV System Orientations
7.3.8.3 Correlation with Load
7.3.8.4 Demand Side Management (DSM) Approach
7.3.8.5 On Load Tap Changer Transformer (OLTC)
7.3.8.6 Storage
7.3.8.7 Reactive Power Control (RPC)
7.3.9 Summary
7.4 Characteristics of Different Smart Inverter Functions
7.4.1 Constant Power Factor
7.4.1.1 Advantages
7.4.1.2 Potential Issues
7.4.2 Volt–Var Function
7.4.2.1 Advantages
7.4.2.2 Potential Issues
7.4.3 Volt–Watt Control
7.4.3.1 Advantages
7.4.3.2 Potential Issues
7.4.4 Active Power Limit
7.4.4.1 Advantages
7.4.4.2 Potential Issues
7.5 Factors Affecting Hosting Capacity of Distribution Feeders
7.5.1 Size and Location of DER
7.5.2 Physical Characteristics of Distribution System
7.5.3 DER Technology
7.5.4 PV Hosting Capacity Estimation
7.5.4.1 Impact of Feeder Characteristics
7.5.4.2 Impact of Smart Inverter Functions
7.6 Determination of Settings of Constant Power Factor Function
7.6.1 Single DER System
7.6.2 Multiple DERs
7.6.2.1 Median Feeder X/R Ratio
7.6.2.2 Weighted Average X/R Ratio
7.6.2.3 Sensitivity Analysis Based Technique
7.6.2.4 Performance Comparison of the Three Methods
Median Feeder X/R Ratio
Weighted Average X/R Ratio
Sensitivity Analysis Based Technique
7.7 Impact of DER Interconnection Transformer
7.8 Determination of Smart Inverter Function Settings from Quasi‐Static Time‐Series (QSTS) Analysis
7.8.1 Development of Detailed Feeder Model
7.8.1.1 Distribution System
7.8.1.2 Conventional Voltage Regulation Equipment
7.8.1.3 PV Systems with Smart Inverter Controls
7.8.1.4 Type of Smart Inverter Control
7.8.1.5 Performance Criteria
7.8.2 Simulation of Quasi‐Static Time‐Series Model
7.8.2.1 Characterization of Solar Conditions
7.8.2.2 Characterization of Load Conditions
7.8.2.3 Simulation Studies
7.8.3 Analysis of Results
7.8.4 Selection of Appropriate Setting
7.9 Guidelines for Selection of Smart Inverter Settings
7.9.1 Autonomous Default Settings
7.9.2 Non‐optimized Settings
7.9.3 Optimized Settings
7.10 Determination of Sites for Implementing DERs with Smart Inverter Functions
7.11 Mitigation Methods for Increasing Hosting Capacity
7.12 Increasing Hosting Capacity in Thermally Constrained Distribution Networks
7.13 Utility Simulation Studies of Smart Inverters for Increasing Hosting Capacity. 7.13.1 Voltage Control in a Distribution Feeder with High PV Penetration
Key Takeaways
7.13.2 Smart Inverter Functions for Increasing Hosting Capacity in New York Distribution Systems
Key Takeaways
7.13.3 Impact of Different Smart Inverter Functions in Increasing Hosting Capacity in Hawaii
Key Takeaways
7.13.4 Smart Inverter Impacts on California Distribution Feeders with Increasing PV Penetration
7.13.4.1 Methodology
7.13.4.2 Voltage Profile Along Feeder
7.13.4.3 Maximum Voltage
7.13.4.4 Minimum Voltage
7.13.4.5 Tap Operations
7.13.4.6 Line Losses
7.13.4.7 Voltage Fluctuations
Key Takeaways
7.13.5 Mitigation Methods to Increase Feeder Hosting Capacity
7.13.5.1 Assessment of Base Feeder
7.13.5.2 Integration Solution Assessment
7.13.5.3 Volt–Watt Function
7.13.5.4 Volt–Var Function
7.13.5.5 Watt‐Power Factor Function
7.13.5.6 Fixed Power Factor Function
Key Takeaways
7.13.6 Impact of Smart Inverter Functions in Increasing Hosting Capacity in Five California Distribution Feeders
7.13.6.1 Volt–Var Control
7.13.6.2 Volt–Watt Control
7.13.6.3 Limiting Maximum Real Power (LMRP) Output Function
7.13.6.4 Selection of the Smart Inverter Settings
Key Takeaways
7.13.7 Hosting Capacity Experience in Utah
7.13.7.1 Constant Power Factor Function
7.13.7.2 Volt–Var Function
Key Takeaways
7.14 Field Implementation of Smart Inverters for Increasing Hosting Capacity
7.14.1 Voltage Control by Constant Power Factor Function in a Distribution Feeder in Fontana, USA
Key Takeaways
7.14.2 Smart Inverter Demonstration in Porterville Feeder in California
Key Takeaways
7.14.3 Improvement in Feeder Hosting Capacity Through Smart Inverter Controls in Upper Austria
Key Takeaways
7.14.4 Demonstration of Smart Inverter Controls under the META PV Project Funded by the European Commission
Key Takeaways
7.14.5 Arizona Public Service Solar Partner Program
Key Takeaways
7.14.6 Increasing Renewables Hosting Capacity in the Czech Republic
7.14.6.1 Use Case 1: Increasing DER Hosting Capacity of LV Distribution Networks
7.14.6.2 Use Case 2: Increasing DER Hosting Capacity in MV Networks
Key Takeaways
7.14.7 Hosting Capacity Experience in Salt River Project
Key Takeaways
7.15 Conclusions
References
8 CONTROL COORDINATION OF SMART PV INVERTERS
8.1 Concepts of Control Coordination. 8.1.1 Need for Coordination
8.1.2 Frequency Range of Control Interactions
8.1.2.1 Steady‐State Interactions
8.1.2.2 Electromechanical Oscillation Interactions
8.1.2.3 Control System Interactions
8.1.2.4 Subsynchronous Oscillation (SSO) Interactions
8.1.2.5 High‐frequency Interactions
8.1.3 Principle of Coordination
8.2 Coordination of Smart Inverters with Conventional Voltage Controllers
8.2.1 European IGREENGrid Project
8.2.2 Interaction of Smart Inverters with Load Tap Changing Transformers
8.2.2.1 System Modeling
8.2.2.2 Simulation Studies
8.2.3 Coordination of Smart Inverters with Distribution Voltage Control Strategies
8.2.3.1 System Modeling. Load
Load Tap Changer
PV Systems
Volt–Var Smart Inverter Functions
8.2.3.2 Simulation Studies
8.2.4 Coordination of Transformer On‐Load Tap Changer and PV Smart Inverters for Voltage Control of Distribution Feeders
8.3 Control Interactions – Lessons Learned from Coordination of FACTS Controllers for Voltage Control
8.3.1 Controller Interaction Among Static Var Compensators in a Test System
8.3.2 Controller Interaction Among Multiple Static Var Compensators in Hydro‐Quebec System
8.4 Control Interactions Among Smart PV Inverters and their Mitigation
8.4.1 Concepts of Control Stability with Volt–Var Control in a Single Smart PV Inverter
8.4.2 Concepts of Control Stability with Volt–Var Control with Multiple Smart PV Inverters
8.4.3 Control Interactions Within a Smart Inverter
8.4.4 Smart Inverters with Volt–Var Functions
8.4.5 Control Interaction Between Volt–Var Controls of Smart Inverters
8.4.6 Oscillations Due to Voltage Control by Smart Inverter
8.4.7 Control Interactions of Volt–Var Controllers
8.4.8 Controller Interaction Between Volt–Var and Volt–Watt Controllers
8.5 Study of Smart Inverter Controller Interactions
8.6 Case Study of Controller Coordination of Smart Inverters in a Realistic Distribution System
8.6.1 Study System
8.6.2 Small Signal Modeling of Study System
8.6.2.1 Network
8.6.2.2 PV Plant
PV Power Circuit
PV Control System
8.6.2.3 Overall Study System Model
8.6.3 Small Signal Studies
8.6.3.1 Impact of Delay
8.6.3.2 Impact of Response Time
8.6.4 Time Domain Simulation Studies
8.6.4.1 Impact of Delay
8.6.4.2 Impact of Response Time
8.6.5 Summary
8.7 Control Coordination of PV‐STATCOM and DFIG Wind Farm for Mitigation of Subsynchronous Oscillations
8.7.1 Study System
8.7.2 Control System of DFIG
8.7.3 Control System of PV‐STATCOM
8.7.4 Optimization of Subsynchronous Damping Controllers
8.7.5 System Response with No Subsynchronous Damping Controls
8.7.6 Independently Optimized SSDC of DFIG Converter
8.7.7 Independently Optimized SSDC of PV‐STATCOM
8.7.8 Uncoordinated SSDCs of PV‐STATCOM and DFIG Converter
8.7.9 Coordinated SSDCs of PV‐STATCOM and DFIG Converter
8.8 Control Interactions Among Plants of Inverter Based Resources and FACTS/HVDC Controllers
8.9 Conclusions
References
9 EMERGING TRENDS WITH SMART SOLAR PV INVERTERS
9.1 Combination of Smart PV Inverters with Battery Energy Storage Systems (BESS)
9.1.1 Increasing Hosting Capacity
9.1.2 Capacity Firming
9.1.3 Preventing Curtailment of Wind/Solar Plant Outputs and Managing Ramp Rates
9.2 Combination of Smart PV Inverters with Electric Vehicle Charging Systems
9.3 Combination of Smart PV Inverters with Battery Energy Storage Systems (BESS) and EV Charging Systems
Energy Savings Through CVR and Line Loss Reduction
9.4 Grid Forming Inverter Technology
9.4.1 Grid Following Inverters
9.4.2 Grid Forming Inverters
9.4.3 Considerations in the Application of Grid Forming Inverters
9.5 Field Demonstrations of Smart Solar PV Inverters
9.5.2 Reactive Power Control by Solar PV Plant in China
9.5.2 Active Power Controls by a PV Plant in Puerto Rico Island Grid, USA
Fast Frequency Response
9.5.3 Reliability Services by a 300 MW Solar PV Plant in California, USA
9.5.3.1 Automatic Generation Control (AGC) Test
9.5.3.2 Droop Test During Underfrequency Event
9.5.3.3 Droop Test During Overfrequency Event
9.5.3.4 Power Factor Control Test
9.5.4 Night and Day PV-STATCOM Operation by a Solar PV Plant in Ontario, Canada
9.5.4.1 Study System
9.5.4.2 PV‐STATCOM Controller
9.5.4.3 Response of Conventional PV Inverter to a Large Disturbance During Daytime
9.5.4.4 Response of PV‐STATCOM to a Large Disturbance During Daytime
9.5.4.5 Response of Conventional Inverter to a Large Disturbance During Nighttime
9.5.4.6 Response of PV‐STATCOM to a Large Disturbance During Nighttime
9.5.4.7 Summary
9.5.5 Nighttime Reactive Power Support from a Solar PV Plant in UK
9.5.6 Commercial Ancillary Grid Services by Solar PV Plant in Chile
9.5.7 Nighttime Reactive Power Control by a Solar PV Plant in India
9.6 Potential of New Revenue Making Opportunities for Smart Solar PV Inverters
9.6.1 Providing Ancillary Services Without Curtailing PV Power Output
9.6.2 Providing Ancillary Services by Curtailing PV Power Output. 9.6.2.1 Fast Frequency Response and Frequency Regulation Services
9.6.2.2 Flexible Solar Operation
9.6.3 Providing Reactive Power Support at Night
9.6.4 Providing STATCOM Functionalities
9.7 Conclusions
References
INDEX. a
c
d
f
g
h
i
l
m
n
p
r
s
t
v
w
WILEY END USER LICENSE AGREEMENT
Отрывок из книги
IEEE Press 445 Hoes Lane Piscataway, NJ 08854
.....
Despite the gradual emergence of accurate forecasting, the power system must still respond and adapt to the changing power output from such IBRs and DERs so as not to impact system reliability and continuity of the power supply to customers.
Increasing solar generation reduces the net load especially during periods of relatively low loads and high generation such as in Spring. CAISO has produced a plot of “net load” which is the difference between forecasted load and expected electricity production from variable generation sources such as solar PV systems. In certain times of the year, these curves produce a “belly” like appearance in the mid‐afternoon that quickly ramps up to produce an “arch” similar to the neck of a duck – hence the popular industry given name “The Duck Chart” shown in Figure 1.13 [51]. The more solar generation increases, the more the curve looks like the belly of a duck. This problem mainly occurs during springtime when the weather is sunny but still cool. At this time, solar generation is high but electricity demand is less as air‐conditioning loads are low [52].
.....