Soft-Switching Technology for Three-phase Power Electronics Converters
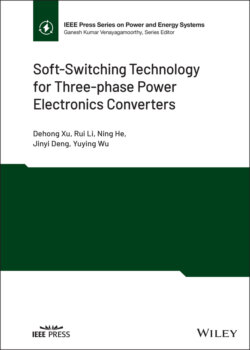
Реклама. ООО «ЛитРес», ИНН: 7719571260.
Оглавление
Rui Li. Soft-Switching Technology for Three-phase Power Electronics Converters
Table of Contents
List of Tables
List of Illustrations
Guide
Pages
Soft‐Switching Technology for Three‐phase Power Electronics Converters
Preface
Nomenclature. Subscripts
Superscripts
Variables
1 Introduction
1.1 Requirement of Three‐phase Power Conversions
1.1.1 Three‐phase Converters
1.1.2 Switching Frequency vs. Conversion Efficiency and Power Density
1.1.3 Switching Frequency and Impact of Soft‐switching Technology
1.2 Concept of Soft‐switching Technique
1.2.1 Soft‐switching Types
1.2.2 Soft‐switching Technique for Three‐phase Converters
1.3 Applications of Soft‐switching to Three‐phase Converters. 1.3.1 Renewable Energy and Power Generation
1.3.2 Energy Storage Systems
1.3.3 Distributed FACTS Devices
1.3.4 Uninterruptible Power Supply
1.3.5 Motor Drives
1.3.6 Fast EV Chargers
1.3.7 Power Supply
1.4 The Topics of This Book
References
2 Basics of Soft‐switching Three‐phase Converters
2.1 Introduction
2.2 Switching Characteristics of Three‐phase Converters
2.2.1 Control of Three‐phase Converters
2.2.2 Switching Transient Process and Switching Loss
2.2.3 Diode Turn‐off and Reverse Recovery
2.2.4 Stray Inductance on Switching Process
2.2.5 Snubber
2.3 Classification of Soft‐switching Three‐phase Converters
2.4 DC‐side Resonance Converters
2.4.1 Resonant DC‐link Converters
2.4.2 Active‐clamped Resonant DC‐link (ACRDCL) Converter
2.4.3 ZVS‐SVM Active‐clamping Three‐phase Converter. 2.4.3.1 Active‐clamping DC–DC Converter
2.4.3.2 Active‐clamping Three‐phase Converter
2.5 AC‐side Resonance Converters
2.5.1 Auxiliary Resonant Commutated Pole Converter
2.5.2 Coupled‐inductor Zero Voltage‐transition (ZVT) Inverter
2.5.3 Zero‐current Transition (ZCT) Inverter
2.6 Soft‐switching Inverter with TCM Control
2.7 Summary
References
3 Soft‐switching PWM Control for Active Clamped Three‐phase Converters
3.1 Introduction
3.2 PWM of Three‐phase Converters
3.3 Edge‐aligned PWM
3.4 ZVS Active‐clamping Converter with Edge‐aligned PWM
3.4.1 Stage Analysis
3.4.2 ZVS Conditions
3.4.2.1 The First Resonant Stage
3.4.2.2 The Second Resonant Stage
3.4.2.3 Steady Conditions
3.4.3 Impact of PWM Scheme and Load on ZVS Condition
3.5 Control Diagram of the Converter with EA‐PWM
3.6 ZVS‐SVM
3.6.1 Vector Sequence
3.6.2 ZVS‐SVM Scheme
3.6.3 Characteristics of the Converter with ZVS‐SVM
3.7 Summary
References
4 Three‐phase Rectifier with Compound Active‐clamping Circuit
4.1 Introduction
4.2 Operation Principle of CAC Rectifier
4.2.1 Space Vector of Three‐phase Grid Voltage
4.2.2 Space Vector Modulation of Three‐phase Converter
4.2.3 Switching Scheme of CAC Rectifier
4.3 Circuit Analysis
4.3.1 Operation Stage Analysis
4.3.2 Resonant Stages Analysis
4.3.3 Steady State Analysis
4.3.4 Soft‐switching Condition
4.3.5 Control Technique of Compound Active‐clamping Three‐phase Rectifier
4.4 Prototype Design
4.4.1 Specifications of a 40 kW Rectifier
4.4.2 Parameter Design
4.4.3 Experiment Platform and Testing Results
4.5 Summary
References
5 Three‐phase Rectifier with Minimum Voltage Active‐clamping Circuit
5.1 Introduction
5.2 Operation Principle of MVAC Rectifier. 5.2.1 Space Vector Modulation of Three‐phase Converter
5.2.2 Switching Scheme of MVAC Rectifier
5.3 Circuit Analysis of MVAC Rectifier. 5.3.1 Operation Stage Analysis
5.3.2 Resonant Stages Analysis
5.3.3 Steady State Analysis
5.3.4 Soft‐switching Condition
5.3.5 Control Technique of Minimum Voltage Active‐clamping Three‐phase Rectifier
5.4 Prototype Design
5.4.1 Specifications of a 30 kW Rectifier
5.4.2 Parameter Design
5.4.3 Experiment Platform and Testing Results
5.5 Summary
References
6 Three‐phase Grid Inverter with Minimum Voltage Active‐clamping Circuit
6.1 Introduction
6.2 Operation Principle of MVAC Inverter. 6.2.1 Space Vector of Three‐phase Grid Voltage
6.2.2 Space Vector Modulation of Three‐phase Inverter
6.2.3 Switching Scheme of MVAC Inverter Under Unit Power Factor
6.2.4 Generalized Space Vector Modulation Method of MVAC Inverter with Arbitrary Output
6.3 Circuit Analysis
6.3.1 Operation Stage Analysis
6.3.2 Resonant Stages Analysis
6.3.3 Steady‐state Analysis
6.3.4 Soft‐switching Condition
6.3.5 Control Technique of MVAC Inverter
6.4 Design Prototype
6.4.1 Specifications of a 30‐kW Inverter
6.4.2 Parameter Design
6.4.3 Experiment Results
6.5 Summary
References
7 Three‐phase Inverter with Compound Active‐clamping Circuit
7.1 Introduction
7.2 Scheme of ZVS‐SVM. 7.2.1 Switch Commutations in Main Bridges of Three‐phase Inverter
7.2.2 Derivation of ZVS‐SVM
7.3 Circuit Analysis
7.3.1 Operation Stage Analysis
7.3.2 Resonant Stages Analysis
7.3.3 Steady‐state Analysis
7.3.4 Soft‐switching Condition
7.3.5 Resonant Time Comparison
7.4 Implementation of ZVS‐SVM. 7.4.1 Regulation of Short Circuit Stage
7.4.2 Implementation in Digital Controller
7.4.3 Control Block Diagram with ZVS‐SVM
7.5 Prototype Design. 7.5.1 Specifications of a 30‐kW Inverter
7.5.2 Parameter Design
7.5.2.1 Requirement of Diode Reverse Recovery Suppression
7.5.2.2 Requirement of Voltage Stress
7.5.2.3 Requirement of reducing turn‐off loss in auxiliary switch
7.5.2.4 Requirement of Minimum Resonant Capacitance
7.5.2.5 Requirement of Resonant Time
7.5.3 Experiment Platform and Testing Results
7.6 Summary
References
8 Loss Analysis and Optimization of a Zero‐voltage‐switching Inverter
8.1 Introduction
8.2 Basic Operation Principle of the CAC ZVS Inverter
8.2.1 Operation Stage Analysis
8.2.2 ZVS Condition Derivation
8.3 Loss and Dimension Models
8.3.1 Loss Model of IGBT Devices. 8.3.1.1 Conduction Loss of IGBT Devices
8.3.1.2 Switching Loss of the IGBT Devices
8.3.2 Loss and Dimension Models of Resonant Inductor
8.3.3 Loss and Dimension Models of the Filter Inductor
8.3.4 Dimension Model of Other Components. 8.3.4.1 Clamping Capacitor
8.3.4.2 Heat Sink
8.4 Parameters Optimization and Design Methodology
8.4.1 Objective Function
8.4.2 Constrained Conditions
8.4.3 Optimization Design
8.5 Prototype and Experimental Results
8.6 Summary
References
9 Design of the Resonant Inductor
9.1 Introduction
9.2 Fundamental of Inductor
9.3 Design Methodology
9.3.1 Cross‐section Area of the Core Ac
9.3.2 Window Area Ae
9.3.3 Area‐product Ap
9.3.4 Turns of Winding N
9.3.5 Length of the Air Gap lg
9.3.6 Winding Loss Pdc
9.3.7 Core Loss Pcore
9.3.8 Design Procedure
9.4 Design Example
9.4.1 Barrel Winding Discussion
9.4.1.1 Winding Position Discussion
9.4.1.2 Winding Thickness Discussion
9.4.2 Flat Winding Discussion
9.4.2.1 Different Structures Comparison
9.4.2.2 Winding Position Discussion
9.5 Design Verification
9.5.1 Simulation Verification
9.5.2 Experimental Verification
9.6 Summary
References
10 Soft‐switching SiC Three‐phase Grid Inverter
10.1 Introduction
10.2 Soft‐switching Three‐phase Inverter. 10.2.1 SVM Scheme in Hard‐switching Inverter
10.2.2 ZVS‐SVM Scheme in Soft‐switching Inverter
10.2.3 Operation Stages and ZVS Condition of Soft‐switching Inverter. 10.2.3.1 Operation Stages Analysis
10.2.3.2 ZVS Condition Derivation. 10.2.3.2.1 Resonant Stages Analysis
10.2.3.2.2 Steady State Analysis
10.2.3.2.3 Soft‐Switching Condition
10.3 Efficiency Comparison of Hard‐switching SiC Inverter and Soft‐switching SiC Inverter
10.3.1 Parameters Design of Soft‐switching SiC Inverter
10.3.1.1 AC Filter Inductor
10.3.1.2 Resonant Parameters
10.3.1.2.1 Requirement of Voltage Stress
10.3.1.2.2 Requirement of the Current Stress in Main Switches and Auxiliary Switch
10.3.1.2.3 Requirement of Resonant Time
10.3.1.2.4 Requirement of Minimum Resonant Capacitance
10.3.1.3 DC Filter Capacitor
10.3.1.4 Clamping Capacitor
10.3.1.5 Cores Selection
10.3.1.6 Switching Loss Measurement
10.3.2 Comparison of Two SiC Inverters
10.3.2.1 Loss Distributions
10.3.2.2 Efficiency Stiffness
10.3.2.3 Passive Components Volumes
10.3.3 Experimental Verification
10.3.3.1 Efficiency Test
10.3.3.2 Passive Components Volumes Comparison
10.4 Design of Low Stray Inductance Layout in Soft‐switching SiC Inverter. 10.4.1 Oscillation Model
10.4.2 Design of Low Stray Inductance 7‐in‐1 SiC Power Module
10.4.3 7‐in‐1 SiC Power Module Prototype and Testing Results. 10.4.3.1 Stray Inductance Measurement
10.4.3.2 Voltage Stress Comparison
10.5 Design of Low Loss Resonant Inductor in Soft‐switching SiC Inverter
10.5.1 Impact of Distributed Air Gap
10.5.2 Optimal Flux Density Investigation
10.5.3 Optimal Winding Foil Thickness Investigation
10.5.4 Resonant Inductor Prototypes and Loss Measurement
10.6 Summary
References
11 Soft‐switching SiC Single‐phase Grid Inverter with Active Power Decoupling
11.1 Introduction
11.1.1 Modulation Methods for Single‐phase Inverter
11.1.2 APD in Single‐phase Grid Inverter
11.2 Operation Principle
11.2.1 Topology and Switching Scheme
11.2.2 Stage Analysis
11.3 Circuit Analysis
11.3.1 Resonant Stages Analysis
11.3.2 Steady‐state Analysis
11.3.3 Soft‐switching Condition
11.3.4 Short Circuit Current
11.4 Design Prototype. 11.4.1 Rated Parameters of a 1.5‐kW Inverter
11.4.2 Parameter Design
11.4.3 Experimental Platform and Testing Results
11.5 Summary
References
12 Soft‐switching SiC Three‐phase Four‐wire Back‐to‐back Converter
12.1 Introduction
12.2 Operation Principle
12.2.1 Commutations Analysis
12.2.2 Operation Scheme
12.2.3 Stage Analysis
12.3 Circuit Analysis
12.3.1 Resonant Stage Analysis
12.3.2 Steady State Analysis
12.3.3 ZVS Condition
12.4 Design Prototype
12.4.1 Parameters Design
12.4.2 Loss Analysis
12.4.3 Experimental Results
12.5 Summary
References
Appendix
A.1 Basic of SVM
A.2 Switching Patterns of SVM 12
A.3 Switching Patterns of ZVS‐SVM
A.4 Inverter Loss Models
A.4.1 Loss Model of Hard‐switching Three‐phase Grid Inverter
A.4.1.1 Conducting Loss
A.4.1.2 Switching Loss
A.4.1.3 AC Filter Inductor Loss and Volume Estimations
A.4.2 Loss Model of Soft‐switching Three‐phase Grid Inverter
A.4.2.1 Loss in Main Switches
A.4.2.2 Loss in Auxiliary Switch
A.4.2.3 Loss and Volume of Filter Inductor and Resonant Inductor
A.5 AC Filter Inductance Calculation
A.6 DC Filter Capacitance Calculation
Index. a
b
c
d
e
f
g
h
i
k
l
m
n
o
p
q
r
s
t
u
v
w
z
Books in the IEEE Press Series on Power and Energy Systems
WILEY END USER LICENSE AGREEMENT
Отрывок из книги
IEEE Press 445 Hoes Lane Piscataway, NJ 08854
.....
In the DC‐side resonance converters, an auxiliary resonant circuit is installed between DC input source and DC side of three‐phase switch bridge of the converter. The fundamental philosophy of the DC‐side resonance is to use an auxiliary resonant circuit to create zero‐voltage duration at the DC side of the three‐phase switch bridge at the desired switching instant. Thus all devices of the switch bridge are turned on or turned off when the voltage on them is equal to zero so that both turn‐on loss and turn‐off loss are significantly reduced. Besides, the DC‐side resonance converter only needs one auxiliary resonant circuit regardless of the number of AC phases of the converter. This simple structure makes DC‐side resonance attractive in multiphase converter applications. The resonant DC link (RDCL) converter [5] is milestone topology in evolution of soft‐switching history. To reduce voltage stress on the devices, a revised version known as active clamped RDCL (ACRDCL) converter occurred [6, 7]. Both RDCL and ACRDCL converters are controlled with discrete pulse modulation (DPM). It is found that the soft‐switching converters with DPM require higher switching frequency than that of the PWM converter for comparable current spectral performance. Many other topologies have been developed such as the quasi‐resonant DC link (QRDCL) PWM inverter with PWM control [8–11]. They often use more complex auxiliary circuit. Zero‐voltage‐switching SVM (ZVS‐SVM) for three‐phase active clamping converters was proposed by Dehong Xu [13, 14]. The auxiliary power device only switches once in each switching cycle to realize ZVS for all the switches. It features fixed switching frequency and lower voltage stress of the power switch devices. The converter basically operates like PWM converter [15, 16]. Afterward it is generalized to edge‐aligned PWM (EA‐PWM) [17–19]. EA‐PWM is suitable to three‐phase converter, three‐phase four‐wire converter, three‐phase four‐wire BTB converter, etc.
The second class of the soft‐switching converter is AC resonance converters. Auxiliary resonant circuits are installed in AC side of the switch bridge. Distinctive advantage of the AC‐side resonance is that the auxiliary circuits are in shunt with the switch bridge and does not carry the load current. Thus the conduction loss in the auxiliary circuits is smaller. In addition, SPWM and SVM control can be applied because the converters basically operate as conventional PWM converters. Auxiliary resonant commutated pole (ARCP) converter is one of the earliest AC resonance converters [12, 20]. It achieves ZVS‐on for main switches and ZCS‐off for auxiliary switches. The inductor coupled zero‐voltage transition (ZVT) inverter achieves ZVS‐on for main switches and near‐zero current turn off for auxiliary switches [21]. DC‐side split capacitor voltage control needed for ARCP converter is avoided. The zero‐current transition (ZCT) inverter achieves zero current switching for all of the main and auxiliary switches and their antiparallel diodes [22, 23]. It is suitable to converters with IGBT devices, which can reduce turn‐off loss of IGBT due to its tail current. Other AC resonance circuits are developed [24–27]. The AC resonance converter has complex circuit because it generally needs three auxiliary resonant circuits. The number of power devices to be controlled are almost doubled in comparison to the original converter.
.....