Geochemistry
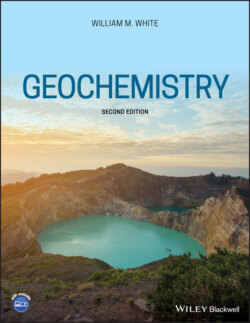
Реклама. ООО «ЛитРес», ИНН: 7719571260.
Оглавление
William M. White. Geochemistry
Table of Contents
List of Tables
List of Illustrations
Guide
Pages
Geochemistry
Preface
About the companion website
Chapter 1 Introduction. 1.1 INTRODUCTION
1.2 BEGINNINGS
1.3 GEOCHEMISTRY IN THE TWENTY-FIRST CENTURY
1.4 THE PHILOSOPHY OF SCIENCE
1.4.1 Building scientific understanding
1.4.2 The scientist as skeptic
1.5 ELEMENTS, ATOMS, CRYSTALS, AND CHEMICAL BONDS: SOME CHEMICAL FUNDAMENTALS. 1.5.1 The periodic table
1.5.2 Electrons and orbits
1.5.3 Some chemical properties of the elements
1.5.4 Chemical bonding. 1.5.4.1 Covalent, ionic, and metal bonds
1.5.4.2 Van der Waals interactions and hydrogen bonds
1.5.5 Molecules, crystals, and minerals. 1.5.5.1 Molecules
1.5.5.2 Crystals
1.6 A BRIEF LOOK AT THE EARTH. 1.6.1 Structure of the Earth
1.6.2 Plate tectonics and the hydrologic cycle
1.7 A LOOK AHEAD
REFERENCES AND SUGGESTIONS FOR FURTHER READING
NOTES
Chapter 2 Energy, entropy, and fundamental thermodynamic concepts. 2.1 THE THERMODYNAMIC PERSPECTIVE
2.2 THERMODYNAMIC SYSTEMS AND EQUILIBRIUM
2.2.1 Fundamental thermodynamic variables
2.2.2 Properties of state
2.3 EQUATIONS OF STATE
2.3.1 Ideal gas law
2.3.2 Equations of state for real gases. 2.3.2.1 Van der Waals equation
2.3.2.2 Other equations of state for gases
2.3.3 Equation of state for other substances
2.4 TEMPERATURE, ABSOLUTE ZERO, AND THE ZEROTH LAW OF THERMODYNAMICS
2.5 ENERGY AND THE FIRST LAW OF THERMODYNAMICS. 2.5.1 Energy
2.5.2 Work
2.5.3 Path independence, exact differentials, state functions, and the first law
2.6 THE SECOND LAW AND ENTROPY. 2.6.1 Statement
2.6.2 Statistical mechanics: a microscopic perspective of entropy
2.6.2.1 Microscopic interpretation of temperature
2.6.2.2 Entropy and volume
2.6.2.3 Summary
2.6.3 Integrating factors and exact differentials
Example 2.1 Entropy in reversible and irreversible reactions
2.7 ENTHALPY
Example 2.2 Measuring enthalpies of reaction
2.8 HEAT CAPACITY
2.8.1 Constant volume heat capacity
2.8.2 Constant pressure heat capacity
2.8.3 Energy associated with volume and the relationship between Cv and Cp
2.8.4 Heat capacity of solids: a problem in quantum physics
2.8.4.1 The Boltzmann distribution law
2.8.4.2 The partition function
2.8.4.3 Energy distribution in solids
2.8.5 Relationship of entropy to other state variables
2.8.6 Additive nature of silicate heat capacities
2.9 THE THIRD LAW AND ABSOLUTE ENTROPY. 2.9.1 Statement of the third law
2.9.2 Absolute entropy
Example 2.3 Configurational entropy
2.10 CALCULATING ENTHALPY AND ENTROPY CHANGES. 2.10.1 Enthalpy changes due to changes in temperature and pressure
Example 2.4 Calculating isobaric enthalpy changes
2.10.2 Changes in enthalpy due to reactions and change of state
Example 2.5 Enthalpies (or heats) of reaction and Hess's law
2.10.3 Entropies of reaction
Example 2.6 Calculating enthalpy and entropy changes
2.11 FREE ENERGY
2.11.1 Helmholtz free energy
2.11.2 Gibbs free energy. 2.11.2.1 Derivation
2.11.2.2 Gibbs free energy change in reactions
2.11.3 Criteria for equilibrium and spontaneity
2.11.4 Temperature and pressure dependence of the Gibbs free energy
Example 2.7 Using Gibbs free energy to predict equilibrium
Example 2.8 Predicting the equilibrium pressure of a mineral assemblage
Example 2.9 Volume and free energy changes for finite compressibility
2.12 THE MAXWELL RELATIONS*
2.13 SUMMARY
REFERENCES AND SUGGESTIONS FOR FURTHER READING
PROBLEMS
NOTES
Chapter 3 Solutions and thermodynamics of multicomponent systems. 3.1 INTRODUCTION
3.2 PHASE EQUILIBRIA. 3.2.1 Some definitions. 3.2.1.1 Phase
3.2.1.2 Species
3.2.1.3 Component
3.2.1.4 Degrees of freedom
3.2.2 The Gibbs phase rule
3.2.3 The Clapeyron equation
Example 3.1 The graphite–diamond transition
3.3 SOLUTIONS
3.3.1 Raoult's law
3.3.2 Henry's law
3.4 CHEMICAL POTENTIAL. 3.4.1 Partial molar quantities
3.4.2 Definition of chemical potential and relationship to Gibbs free energy
3.4.3 Properties of the chemical potential
3.4.4 The Gibbs–Duhem relation
3.4.5 Derivation of the phase rule
3.5 IDEAL SOLUTIONS
3.5.1 Chemical potential in ideal solutions
3.5.2 Volume, enthalpy, entropy, and free energy changes in ideal solutions
3.6 REAL SOLUTIONS
3.6.1 Chemical potential in real solutions
3.6.2 Fugacities
3.6.3 Activities and activity coefficients
Example 3.2 Using fugacity to calculate Gibbs free energy
3.6.4 Excess functions
Depression of the melting point
3.7 ELECTROLYTE SOLUTIONS
3.7.1 The nature of water and water–electrolyte interaction
3.7.2 Some definitions and conventions
3.7.2.1 Concentration units
3.7.2.2 pH
3.7.2.3 Standard state and other conventions
3.7.3 Activities in electrolytes
3.7.3.1 The Debye–Hückel and Davies equations
3.7.3.2 Limitations to the Debye–Hückel approach
Example 3.3 Calculating activities using the Debye–Hückel equation
3.8 IDEAL SOLUTIONS IN CRYSTALLINE SOLIDS AND THEIR ACTIVITIES
3.8.1 Mixing-on-site model
Example 3.4 Calculating activities using the mixing-on-site model
3.8.2 Local charge balance model
Example 3.5 Activities using the local charge balance model
3.9 EQUILIBRIUM CONSTANTS
3.9.1 Derivation and definition
3.9.2 Law of mass action
Example 3.6 Manipulating reactions and equilibrium constant expressions
3.9.2.1 Le Chatelier's principle
3.9.3 KD values, apparent equilibrium constants, and the solubility product
Example 3.7 Using the solubility product
3.9.4 Henry's law and gas solubilities
3.9.5 Temperature dependence of equilibrium constant
Example 3.8 Calculating equilibrium constants and equilibrium concentrations
3.9.6 Pressure dependence of equilibrium constant
3.10 PRACTICAL APPROACH TO ELECTROLYTE EQUILIBRIUM
3.10.1 Choosing components and species
3.10.2 Mass balance
Example 3.9 Soil organic acid
3.10.3 Electrical neutrality
Example 3.10 Determining the pH of rainwater from its composition
3.10.4 Equilibrium constant expressions
3.11 OXIDATION AND REDUCTION
3.11.1 Redox in aqueous solutions
3.11.1.1 Hydrogen scale potential, EH
Example 3.11 Calculating the EH of net reactions
3.11.1.2 Alternative representation of redox state: pε
3.11.1.3 pε–pH diagrams
Balancing redox reactions for pε–pH diagrams
3.11.2 Redox in magmatic systems
3.12 SUMMARY
REFERENCES AND SUGGESTIONS FOR FURTHER READING
PROBLEMS
NOTES
Chapter 4 Applications of thermodynamics to the Earth. 4.1 INTRODUCTION
4.2 ACTIVITIES IN NONIDEAL SOLID SOLUTIONS. 4.2.1 Mathematical models of real solutions: Margules equations
4.2.1.1 The symmetric solution model
4.2.1.2 The asymmetric solution model
Example 4.1 Computing activities using Margules parameters
4.3 EXSOLUTION PHENOMENA
4.4 THERMODYNAMICS AND PHASE DIAGRAMS
4.4.1 The thermodynamics of melting
Example 4.2 Calculating melting curves
4.4.2 Thermodynamics of phase diagrams for binary systems
4.4.2.1 An example of a simple binary system with complete solution: albite–anorthite
4.4.3 Phase diagrams for multicomponent systems
4.5 GEOTHERMOMETRY AND GEOBAROMETRY
4.5.1 Theoretical considerations
4.5.2 Practical thermobarometers. 4.5.2.1 Univariant reactions and displaced equilibria
4.5.2.2 Solvus equilibria
4.5.2.3 Exchange reactions
Example 4.3 Calculating magma temperatures using the olivine geothermometer
Example 4.4 Using the iron–titanium oxide geothermometer
4.6 THERMODYNAMIC MODELS OF MAGMAS
4.6.1 Structure of silicate melts
4.6.2 Magma solution models
4.6.2.1 The regular solution model of Ghiorso and others: “MELTS”
4.7 REPRISE: THERMODYNAMICS OF ELECTROLYTE SOLUTIONS
4.7.1 Equation of state for water
4.7.2 Activities and mean ionic and single ion quantities
4.7.2.1 Relationship between activity and molality of a salt
4.7.2.2 Mean ionic quantities
Example 4.5 Mean ionic parameters for a fully dissociated electrolyte
4.7.2.3 Single ion properties
4.7.3 Activities in high ionic strength solutions
4.7.3.1 Correction for the concentration of water
4.7.3.2 Effects of solvation
4.7.3.3 Effects of ion association
4.7.3.4 Alternative expressions for activity coefficients
Example 4.6 Activity coefficients in a brine
4.7.3.5 Pitzer equations
Example 4.7 Calculating activity coefficients using PHREEQC
4.7.4 Electrolyte solutions at elevated temperature and pressure. 4.7.4.1 Born equation
4.7.4.2 The HKF model
4.7.4.3 Properties of ore-forming hydrothermal solutions
4.8 SUMMARY
REFERENCES AND SUGGESTIONS FOR FURTHER READING
PROBLEMS
NOTES
Chapter 5 Kinetics: the pace of things. 5.1 INTRODUCTION
5.2 REACTION KINETICS. 5.2.1 Elementary and overall reactions
5.2.2 Reaction mechanisms
5.2.3 Reaction rates
5.2.3.1 The reaction rate for an elementary reaction: composition dependence
5.2.3.2 The reaction rate for an elementary reaction: temperature dependence
5.2.3.3 A general form of the rate equation
Example 5.1 Rate of hydration of CO2(aq)
5.2.4 Rates of complex reactions
Example 5.2 Oxidation of Ferrous Iron
5.2.4.1 Chain reactions and branching
5.2.4.2 Rate-determining step
5.2.5 Steady state and equilibrium
Example 5.3 Racemization of amino acids
5.3 RELATIONSHIPS BETWEEN KINETICS AND THERMODYNAMICS. 5.3.1 Principle of detailed balancing
5.3.2 Enthalpy and activation energy
5.3.3 Aspects of transition state theory
Example 5.4 Estimating ΔG* for the aragonite–calcite transition
Example 5.5 Predicting rates of reversible metamorphic reactions
5.4 DIFFUSION
5.4.1 Diffusion flux and Fick's laws
5.4.1.1 Solutions to Fick's second law
5.4.2 Diffusion in multicomponent systems
Example 5.6 Diffusion in a crystal
Example 5.8 Calculating interdiffusion profiles
5.4.3 Driving force and mechanism of diffusion
5.4.4 Diffusion in solids and the temperature dependence of the diffusion coefficient
5.4.5 Diffusion in liquids
5.4.6 Diffusion in porous media
5.5 SURFACES, INTERFACES, AND INTERFACE PROCESSES
5.5.1 The surface free energy
5.5.2 The Kelvin effect
5.5.3 Nucleation and crystal growth. 5.5.3.1 Nucleation
5.5.3.2 Nucleation rate
Example 5.9 Nucleation of Diopside
5.5.3.3 Heterogeneous nucleation
5.5.3.4 Diffusion-limited and heat-flow limited growth
5.5.3.5 Grain coarsening, static annealing, and Ostwald ripening
5.5.4 Adsorption
5.5.4.1 The relation between concentration and adsorption: Langmuir and Freundlich isotherms
5.5.5 Catalysis
Example 5.10 The Langmuir isotherm
ADSORPTION AND DETERMINATION OF MINERAL SURFACE AREAS
5.6 KINETICS OF DISSOLUTION
5.6.1 Simple oxides
5.6.2 Silicates
5.6.3 Nonsilicates
5.7 DIAGENESIS
5.7.1 Compositional gradients in accumulating sediment
5.7.2 Reduction of sulfate in accumulating sediment
5.8 SUMMARY
REFERENCES AND SUGGESTIONS FOR FURTHER READING
PROBLEMS
NOTES
Chapter 6 Aquatic chemistry. 6.1 INTRODUCTION
6.2 ACID–BASE REACTIONS
6.2.1 Proton accounting, charge balance, and conservation equations. 6.2.1.1 Proton accounting
6.2.1.2 Conservation equations
6.2.1.3 Charge balance
6.2.2 The carbonate system
Example 6.1 Proton, mass, and charge balance equations for Na2CO3 solution
Example 6.2 pH of water in equilibrium with the atmosphere
Example 6.3 pH of a solution with fixed total carbonate concentration
6.2.2.1 Equivalence points
6.2.3 Conservative and nonconservative ions
6.2.4 Total alkalinity and carbonate alkalinity
Example 6.4 The Tableau method of Morel and Hering
6.2.4.1 Alkalinity determination and titration curves
Example 6.5 Calculating alkalinity of spring water
6.2.5 Buffer intensity
6.3 COMPLEXATION
Example 6.6 Calculating buffer intensity
6.3.1 Stability constants
6.3.2 Water-related complexes
Example 6.7 Complexation of Pb
6.3.3 Other complexes
6.3.4 Complexation in fresh waters
Example 6.8 Speciation in fresh water
6.4 DISSOLUTION AND PRECIPITATION REACTIONS. 6.4.1 Calcium carbonate in groundwaters and surface waters
6.4.2 Solubility of Mg
Example 6.9 Calcite solubility in a closed system
Example 6.10 Constructing stability diagrams
6.4.3 Solubility of SiO2
6.4.4 Solubility of Al(OH)3 and other hydroxides
6.4.5 Dissolution of silicates and related minerals
6.5 CLAYS AND THEIR PROPERTIES
6.5.1 Clay mineralogy
6.5.1.1 Kaolinite group (1:1 clays)
6.5.1.2 Pyrophyllite group (2:1 clays)
6.5.1.3 Chlorite group (2:2 clays)
6.5.2 Ion-exchange properties of clays
6.6 MINERAL SURFACES AND THEIR INTERACTION WITH SOLUTIONS
6.6.1 Adsorption
Example 6.11 Adsorption of Pb2+ on hydrous ferric oxide as a function of pH
6.6.2 Development of surface charge and the electric double layer
6.6.2.1 Determination of surface charge
6.6.2.2 Surface potential and the double layer
6.6.2.3 Effect of the surface potential on adsorption
Example 6.12 Effect of surface potential on surface speciation of ferric oxide
6.7 SUMMARY
REFERENCES AND SUGGESTIONS FOR FURTHER READING
PROBLEMS
NOTES
Chapter 7 Trace elements in igneous processes. 7.1 INTRODUCTION
7.1.1 Why care about trace elements?
7.1.2 What is a trace element?
7.2 BEHAVIOR OF THE ELEMENTS. 7.2.1 Goldschmidt's classification
7.2.2 The geochemical periodic table
7.2.2.1 The volatile elements
7.2.2.2 The semivolatiles
7.2.2.3 Alkali and alkaline earth elements
7.2.2.4 The rare earth elements and Y
7.2.2.5 The HFS elements
7.2.2.6 The first series transition metals
7.2.2.7 The noble metals
7.2.2.8 Other elements
7.3 DISTRIBUTION OF TRACE ELEMENTS BETWEEN COEXISTING PHASES. 7.3.1 The partition coefficient
7.3.2 Thermodynamic basis
7.4 FACTORS GOVERNING THE VALUE OF PARTITION COEFFICIENTS. 7.4.1 Temperature and pressure dependence of the partition coefficient
7.4.2 Ionic size and charge
7.4.2.1 Goldschmidt's rules of substitution
7.4.2.2 Quantitative treatment of ionic size and charge
7.4.3 Compositional dependency
Example 7.1 Calculating NBO/T
Example 7.2 Parameterizing partition coefficients
7.4.4 Mineral–liquid partition coefficients for mafic and ultramafic systems
Example 7.3 Calculating partition coefficients
7.5 CRYSTAL-FIELD EFFECTS
7.5.1 Crystal-field theory
7.5.2 Crystal-field influences on transition metal partitioning
7.6 TRACE ELEMENT DISTRIBUTION DURING PARTIAL MELTING
7.6.1 Equilibrium or batch melting
7.6.2 Fractional melting
7.6.3 Zone refining
7.6.4 Multiphase solids
7.6.5 Continuous melting
Example 7.4 Modeling partial melting
7.6.6 Constraints on melting models
7.6.6.1 Relationship between melt fraction and temperature and pressure
7.6.6.2 Mantle permeability and melt distribution and withdrawal
7.6.6.3 Realistic models of mantle melting
7.7 TRACE ELEMENT DISTRIBUTION DURING CRYSTALLIZATION. 7.7.1 Equilibrium crystallization
7.7.2 Fractional crystallization
7.7.3 In situ crystallization
7.7.4 Crystallization in open system magma chambers
7.7.5 Comparing partial melting and crystallization
7.8 SUMMARY OF TRACE ELEMENT VARIATIONS DURING MELTING AND CRYSTALLIZATION
REFERENCES AND SUGGESTIONS FOR FURTHER READING
PROBLEMS
NOTES
Chapter 8 Radiogenic isotope geochemistry. 8.1 INTRODUCTION
8.2 PHYSICS OF THE NUCLEUS AND THE STRUCTURE OF NUCLEI
8.2.1 Nuclear structure and energetics
Example 8.1 Calculating binding energies
8.2.2 The decay of excited and unstable nuclei
8.2.2.1 Gamma decay
8.2.2.2 Alpha decay
8.2.2.3 Beta decay
8.2.2.4 Electron capture
8.2.2.5 Spontaneous fission
MEASURING ISOTOPE RATIOS: MASS SPECTROMETRY
8.3 BASICS OF RADIOGENIC ISOTOPE GEOCHEMISTRY AND GEOCHRONOLOGY
Example 8.2 Calculating isochrons and ages
8.4 DECAY SYSTEMS AND THEIR APPLICATIONS
8.4.1 Rb-Sr
8.4.2 Sm-Nd
Example 8.3 Calculating Nd model ages
8.4.3 Lu-Hf
Example 8.4 Modeling the Sr and Nd isotope evolution of the crust and mantle
8.4.4 Re-Os
8.4.5 La-Ce
8.4.6 U-Th-Pb
U–PB ZIRCON DATING
8.4.7 U and Th decay series isotopes
Example 8.5 Determining the growth rate of a Mn nodule
8.4.8 Isotopes of He and other rare gases. 8.4.8.1 Helium
8.4.8.2 Neon
8.4.8.3 K-Ar-Ca
40AR/39AR DATING
8.5 “EXTINCT” AND COSMOGENIC NUCLIDES. 8.5.1 “Extinct” radionuclides and their daughters
8.5.2 Cosmogenic nuclides
8.5.2.1 14C geochronology
8.5.2.2 36Cl in hydrology
8.5.2.3 Be isotopes
8.5.2.4 Surface exposure ages
8.5.3 Cosmic ray exposure ages of meteorites
8.6 SUMMARY
REFERENCES AND SUGGESTIONS FOR FURTHER READING
PROBLEMS
NOTES
Chapter 9 Stable isotope geochemistry. 9.1 INTRODUCTION
9.1.1 Scope of stable isotope geochemistry
9.1.2 Some definitions. 9.1.2.1 The δ notation
9.1.2.2 The fractionation factor
9.2 THEORETICAL CONSIDERATIONS
9.2.1 Equilibrium isotope fractionations
9.2.1.1 The quantum mechanical origin of isotopic fractionations
9.2.1.2 Predicting isotopic fractionations from statistical mechanics
Example 9.1 Predicting isotopic fractionations
9.2.1.3 Reduced partition function ratios: β factors
9.2.1.4 Temperature dependence of the fractionation factor
9.2.1.5 Composition and pressure dependence
9.2.2 Kinetic isotope fractionations
9.2.3 Mass-dependent and mass-independent fractionations
9.2.4 Isotopic clumping
9.3 ISOTOPE GEOTHERMOMETRY
Example 9.2 Oxygen isotope geothermometry
9.4 ISOTOPIC FRACTIONATION IN THE HYDROLOGIC SYSTEM
9.5 ISOTOPIC FRACTIONATION IN BIOLOGICAL SYSTEMS
9.5.1 Carbon isotope fractionation during photosynthesis
9.5.2 Nitrogen isotope fractionation in biological processes
9.5.3 Oxygen and hydrogen isotope fractionation by plants
9.5.4 Biological fractionation of sulfur isotopes
9.5.5 Isotopes and diet: you are what you eat
9.5.5.1 Isotopes in archaeology
9.5.6 Isotopic “fossils” and the earliest life
9.6 PALEOCLIMATOLOGY
9.6.1 The marine Quaternary δ18O record and Milankovitch cycles
9.6.2 The record in glacial ice
9.6.3 Soils and paleosols
9.7 HYDROTHERMAL SYSTEMS AND ORE DEPOSITS
9.7.1 Water in hydrothermal systems
9.7.2 Water–rock ratios
9.7.3 Sulfur isotopes and ore deposits
9.8 MASS-INDEPENDENT SULFUR ISOTOPE FRACTIONATION AND THE RISE OF ATMOSPHERIC OXYGEN
9.9 STABLE ISOTOPES IN THE MANTLE AND MAGMATIC SYSTEMS. 9.9.1 Stable isotopic composition of the mantle
9.9.1.1 Oxygen
9.9.1.2 Hydrogen
9.9.1.3 Carbon
9.9.1.4 Nitrogen
9.9.1.5 Sulfur
9.9.2 Stable isotopes in crystallizing magmas
9.9.3 Combined fractional crystallization and assimilation
9.10 NONTRADITIONAL STABLE ISOTOPES
9.10.1 Boron isotopes
9.10.2 Li isotopes
9.10.3 Calcium isotopes
9.10.4 Silicon isotopes
9.10.5 Iron isotopes
9.10.6 Mercury isotopes
9.11 SUMMARY
REFERENCES AND SUGGESTIONS FOR FURTHER READING
PROBLEMS
NOTES
Chapter 10 The big picture: cosmochemistry. 10.1 INTRODUCTION
10.2 IN THE BEGINNING … NUCLEOSYNTHESIS. 10.2.1 Astronomical background
10.2.2 The polygenetic hypothesis of Burbidge, Burbidge, Fowler, and Hoyle
10.2.3 Cosmological nucleosynthesis
10.2.4 Nucleosynthesis in stellar interiors. 10.2.4.1 Hydrogen, helium, and carbon burning stars
10.2.4.2 The e-process
10.2.4.3 The s-process
10.2.5 Explosive nucleosynthesis
10.2.5.1 The r-process
10.2.5.2 The p-processes
10.2.6 Nucleosynthesis in interstellar space
10.2.7 Summary
10.3 METEORITES: ESSENTIAL CLUES TO THE BEGINNING
10.3.1 Chondrites: the most primitive objects
10.3.1.1 Chondrite classes and their compositions
10.3.1.2 Chondrules
10.3.1.3 Calcium–aluminum inclusions
10.3.1.4 Amoeboid olivine aggregates
10.3.1.5 The chondrite matrix
10.3.2 Differentiated meteorites
10.3.2.1 Achondrites
10.3.2.2 Irons
10.3.2.3 Stony-irons
10.4 TIME AND THE ISOTOPIC COMPOSITION OF THE SOLAR SYSTEM. 10.4.1 Meteorite ages. 10.4.1.1 Conventional methods
10.4.1.2 Extinct radionuclides
10.4.2 Cosmic ray exposure ages and meteorite parentbodies
10.4.3 Asteroids as meteorite parentbodies
10.4.4 Isotopic anomalies in meteorites. 10.4.4.1 Neon alphabet soup and star dust
10.4.4.2 Isotopic variations in bulk meteorites
10.5 ASTRONOMICAL AND THEORETICAL CONSTRAINTS ON SOLAR SYSTEM FORMATION
10.5.1 Evolution of young stellar objects
10.5.2 The condensation sequence
10.5.3 The solar system
10.5.4 Other solar systems
10.6 BUILDING A HABITABLE SOLAR SYSTEM
10.6.1 Summary of observations
10.6.2 Formation of the planets
10.6.3 Chemistry and history of the Moon
10.6.3.1 Geology and history of the Moon
10.6.3.2 Composition of the Moon
10.6.4 The giant impact hypothesis and formation of the Earth and the Moon
10.6.5 Tungsten isotopes and the age of the Earth
10.7 SUMMARY
REFERENCES AND SUGGESTIONS FOR FURTHER READING
PROBLEMS
NOTES
Chapter 11 Geochemistry of the solid Earth. 11.1 INTRODUCTION
11.2 THE EARTH'S MANTLE
11.2.1 Structure of the mantle and geophysical constraints on mantle composition
11.2.2 Cosmochemical constraints on mantle composition
11.2.3 Observational constraints on mantle composition
11.2.4 Mantle mineralogy and phase transitions. 11.2.4.1 Upper mantle phase changes
11.2.4.2 The transition zone
11.2.4.3 The lower mantle
11.3 ESTIMATING MANTLE AND BULK EARTH COMPOSITION. 11.3.1 Major element composition
11.3.2 Trace element composition
11.3.3 Composition of the bulk silicate earth
11.4 THE EARTH'S CORE AND ITS COMPOSITION. 11.4.1 Geophysical constraints
11.4.2 Cosmochemical constraints
11.4.3 Experimental constraints
11.5 MANTLE GEOCHEMICAL RESERVOIRS
11.5.1 Evidence from oceanic basalts
11.5.2 Evolution of the depleted MORB mantle
11.5.3 Evolution of mantle plume reservoirs
11.5.3.1 The recycling paradigm
11.5.3.2 A primitive component as well
11.5.4 The subcontinental lithospheric mantle
11.6 THE CRUST
11.6.1 The oceanic crust
11.6.1.1 Structure of the oceanic crust
11.6.1.2 Composition of the oceanic crust
11.6.2 The continental crust
11.6.2.1 Composition of the upper continental crust
11.6.2.2 Composition of the middle and lower continental crust
11.6.2.3 Composition of the total continental crust
11.6.3 Growth of the continental crust
11.6.4 Refining the continental crust
11.7 SUBDUCTION ZONE PROCESSES
11.7.1 Major element composition
11.7.2 Trace element composition
11.7.3 Isotopic composition and sediment subduction
11.7.4 Magma genesis in subduction zones
11.8 SUMMARY
REFERENCES AND SUGGESTIONS FOR FURTHER READING
PROBLEMS
NOTES
Chapter 12 Organic geochemistry, the carbon cycle, and climate. 12.1 INTRODUCTION
12.2 A BRIEF BIOLOGICAL BACKGROUND
12.3 ORGANIC COMPOUNDS AND THEIR NOMENCLATURE
12.3.1 Hydrocarbons
12.3.2 Functional groups
12.3.3 Short-hand notations of organic molecules
12.3.4 Biologically important organic compounds
12.3.4.1 Carbohydrates
12.3.4.2 Nitrogen-bearing organic compounds: proteins, nucleotides, and nucleic acids
12.3.4.3 Lipids
12.3.4.4 Lignin and tannins
12.4 THE CHEMISTRY OF LIFE: IMPORTANT BIOCHEMICAL PROCESSES
12.4.1 Photosynthesis
12.4.2 Respiration
12.4.3 The stoichiometry of life
12.5 ORGANIC MATTER IN NATURAL WATERS AND SOILS
12.5.1 Organic matter in soils
12.5.2 Dissolved organic matter in aquatic and marine environments
12.5.3 Hydrocarbons in natural waters
12.6 CHEMICAL PROPERTIES OF ORGANIC MOLECULES. 12.6.1 Acid–base properties
12.6.2 Complexation
Example 12.1 Speciation of organic ligands in fresh water
Example 12.2 Speciation of Cu in fresh water
12.6.3 Adsorption phenomena. 12.6.3.1 The hydrophobic effect and hydrophobic adsorption
12.6.3.2 Other adsorption mechanisms
12.6.3.3 Dependence on pH
12.6.3.4 Role in weathering
12.7 SEDIMENTARY ORGANIC MATTER
12.7.1 Preservation of organic matter
12.7.2 Diagenesis of marine sediments
12.7.3 Diagenesis of aquatic sediments
12.7.4 Summary of diagenetic changes
12.7.5 Biomarkers
12.7.6 Kerogen and bitumen
12.7.6.1 Kerogen classification
12.7.6.2 Bitumen
12.7.7 Isotope composition of sedimentary organic matter. 12.7.7.1 Bulk isotopic composition
12.7.7.2 Compound-specific isotopic analysis
12.8 PETROLEUM AND COAL FORMATION. 12.8.1 Petroleum. 12.8.1.1 Catagenesis and metagenesis
12.8.1.2 Migration and post-generation compositional evolution
12.8.1.3 Composition of crude oils
12.8.2 Compositional evolution of coal
12.9 THE CARBON CYCLE AND CLIMATE
12.9.1 Greenhouse energy balance
12.9.2 The exogenous carbon cycle
12.9.3 The deep carbon cycle
12.9.4 Evolutionary changes affecting the carbon cycle
12.9.5 The carbon cycle and climate through time
12.9.6 Fossil fuels and anthropogenic climate change
12.10 SUMMARY
REFERENCES AND SUGGESTIONS FOR FURTHER READING
PROBLEMS
NOTES
Chapter 13 The land surface: weathering, soils, and streams. 13.1 INTRODUCTION
13.2 REDOX IN NATURAL WATERS
13.2.1 Biogeochemical redox reactions
13.2.2 Eutrophication
13.2.3 Redox buffers and transition metal chemistry
Example 13.1 Redox state of lake water
13.3 WEATHERING, SOILS, AND BIOGEOCHEMICAL CYCLING
13.3.1 Soil profiles
13.3.2 Chemical cycling in soils
13.3.3 Biogeochemical cycling
13.4 WEATHERING RATES
13.4.1 The in situ approach. 13.4.1.1 Weathering profiles
13.4.1.2 Weathering indices
13.4.1.3 Erosion
13.4.2 The watershed approach
13.4.2.1 Watersheds in the Coweeta Basin, Southern Appalachians
Example 13.2 Determining weathering fluxes
13.4.2.2 Thermodynamic and kinetic assessment of stream compositions
13.4.3 Factors controlling weathering rates
13.4.3.1 Lithology
13.4.3.2 Climate: temperature, precipitation, and hydrology
13.4.3.3 Topography and mechanical erosion
13.4.3.4 Role of biota
13.5 THE COMPOSITION OF RIVERS
13.6 CONTINENTAL SALINE WATERS
13.7 SUMMARY
REFERENCES AND SUGGESTIONS FOR FURTHER READING
PROBLEMS
NOTES
Chapter 14 The ocean as a chemical system. 14.1 INTRODUCTION
14.2 SOME BACKGROUND OCEANOGRAPHIC CONCEPTS
14.2.1 Salinity, chlorinity, temperature, and density
14.2.2 Circulation of the ocean and the structure of ocean water
14.2.2.1 Surface circulation
14.2.2.2 Density structure and deep circulation
Example 14.1 Replacement time of deep ocean water
14.3 COMPOSITION OF SEAWATER
14.3.1 Speciation in seawater
Example 14.2 Inorganic complexation of Ni in seawater
14.3.2 Conservative elements
14.3.3 Dissolved gases
14.3.3.1 O2 variation in the ocean
14.3.3.2 Distribution of CO2 in the ocean
14.3.4 Seawater pH and alkalinity
14.3.5 Carbonate dissolution and precipitation
Example 14.3 Calcite solubility in the oceans
14.3.6 Nutrient elements
14.3.7 Particle-reactive elements
14.3.8 One-dimensional advection–diffusion model
Example 14.4 Advection–diffusion model
14.4 SOURCES AND SINKS OF DISSOLVED MATTER IN SEAWATER
14.4.1 Residence time
14.4.2 River and groundwater flux to the oceans
14.4.2.1 Estuaries
14.4.2.2 Submarine groundwater discharge
14.4.3 The hydrothermal flux
14.4.3.1 The composition of hydrothermal fluids
14.4.3.2 Evolution of hydrothermal fluids
14.4.3.3 Hydrothermal fluxes
14.4.4 The atmospheric source
14.4.5 Sedimentary sinks and sources
14.4.5.1 Biogenic sediments
14.4.5.2 Evaporites
14.4.5.3 Red clays, metalliferous sediments, and Mn nodules
14.4.5.4 Porewater fluxes into and out of sediments
14.5 SUMMARY
REFERENCES
PROBLEMS
NOTES
Chapter 15 Applied geochemistry. 15.1 INTRODUCTION
15.2 MINERAL RESOURCES
15.2.1 Ore deposits: definitions and classification. 15.2.1.1 Definitions
15.2.1.2 Classification of ore deposits
15.2.2 Orthomagmatic ore deposits
15.2.3 Hydromagmatic ore deposits
15.2.3.1 Metal solubility in ore forming fluids
15.2.3.2 Porphyry copper deposits
15.2.3.3 Tin alkali granite deposits
15.2.4 Hydrothermal ore deposits. 15.2.4.1 Volcanogenic massive sulfide deposits
15.2.4.2 Stratiform copper deposits
15.2.4.3 Mississippi Valley-type Zn-Pb deposits
15.2.5 Sedimentary ore deposits
15.2.5.1 Banded iron formations
15.2.5.2 Evaporites and brines
15.2.6 Weathering-related ore deposits
15.2.6.1 Bauxites and laterites
15.2.7 Rare earth ore deposits
15.2.8 Geochemical exploration: finding future resources
15.2.8.1 Primary dispersion
15.2.8.2 Secondary dispersion
15.2.8.3 Exploration process
15.3 ENVIRONMENTAL GEOCHEMISTRY
15.3.1 Eutrophication redux
15.3.2 Toxic metals in the environment
15.3.2.1 Mine wastes
15.3.2.2 Atmospheric lead
15.3.2.3 Mercury
15.3.3 Acid deposition
15.4 SUMMARY
REFERENCES
PROBLEMS
NOTES
Appendix Constants, units and conversions. PHYSICAL AND CHEMICAL CONSTANTS
THE EARTH
SI UNITS AND CONVERSIONS
SI PREFIXES
Index
LIST OF EXAMPLES
WILEY END USER LICENSE AGREEMENT
Отрывок из книги
SECOND EDITION
William M. White
.....
Consider a mineral sample, A, in a heat bath, B (B having much more mass than A), and assume they are perfectly isolated from their surroundings. The total energy of the system is fixed, but the energy of A and B will oscillate about their most probable values. The question we ask is what is the probability that system A is in a state such that it has energy EA?
We assume that the number of states accessible to A when it has energy EA is some function of energy:
.....