Computational Methods in Organometallic Catalysis
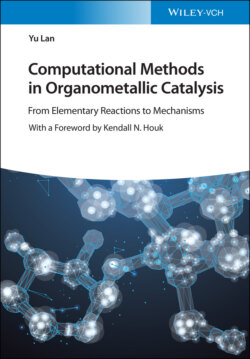
Реклама. ООО «ЛитРес», ИНН: 7719571260.
Оглавление
Yu Lan. Computational Methods in Organometallic Catalysis
Table of Contents
List of Tables
List of Illustrations
Guide
Pages
Computational Methods in Organometallic Catalysis. From Elementary Reactions to Mechanisms
Foreword
Preface
Part I Theoretical View of Organometallic Catalysis
1 Introduction of Computational Organometallic Chemistry
1.1 Overview of Organometallic Chemistry
1.1.1 General View of Organometallic Chemistry
1.1.2 A Brief History of Organometallic Chemistry
1.2 Using Computational Tool to Study the Organometallic Chemistry Mechanism
1.2.1 Mechanism of Transition Metal Catalysis
1.2.2 Mechanistic Study of Transition Metal Catalysis by Theoretical Methods
References
2 Computational Methods in Organometallic Chemistry. 2.1 Introduction of Computational Methods. 2.1.1 The History of Quantum Chemistry Computational Methods
2.1.2 Post‐HF Methods
2.2 Density Functional Theory (DFT) Methods. 2.2.1 Overview of Density Functional Theory Methods
2.2.2 Jacob's Ladder of Density Functionals
2.2.3 The Second Rung in “Jacob's Ladder” of Density Functionals
2.2.4 The Third Rung in “Jacob's Ladder” of Density Functionals
2.2.5 The Fourth Rung in “Jacob's Ladder” of Density Functionals
2.2.6 The Fifth Rung in “Jacob's Ladder” of Density Functionals
2.2.7 Correction of Dispersion Interaction in Organic Systems
2.3 Basis Set and Its Application in Mechanism Studies. 2.3.1 General View of Basis Set
2.3.2 Pople's Basis Sets
2.3.3 Polarization Functions
2.3.4 Diffuse Functions
2.3.5 Correlation‐Consistent Basis Sets
2.3.6 Pseudo Potential Basis Sets
2.4 Solvent Effect
2.5 How to Choose a Method in Computational Organometallic Chemistry. 2.5.1 Why DFT Method Is Chosen
2.5.2 How to Choose a Density Functional
2.5.3 How to Choose a Basis Set
2.6 Revealing a Mechanism for An Organometallic Reaction by Theoretical Calculations
2.7 Overview of Popular Computational Programs
2.8 The Limitation of Current Computational Methods
2.8.1 The Accuracy of DFT Methods
2.8.2 Exact Solvation Effect
2.8.3 Evaluation of Entropy Effect
2.8.4 The Computation of Excited State and High Spin State
2.8.5 Speculation on the Reaction Mechanism
References
3 Elementary Reactions in Organometallic Chemistry
3.1 General View of Elementary Reactions in Organometallic Chemistry
3.2 Coordination and Dissociation. 3.2.1 Coordination Bond and Coordination
3.2.2 Dissociation
3.2.3 Ligand Exchange
3.3 Oxidative Addition
3.3.1 Concerted Oxidative Addition
3.3.2 Substitution‐type Oxidative Addition
3.3.3 Radical‐type Addition
3.3.4 Oxidative Cyclization
3.4 Reductive Elimination
3.4.1 Concerted Reductive Elimination
3.4.2 Substitution‐type Reductive Elimination
3.4.3 Radical‐Substitution‐type Reductive Elimination
3.4.4 Bimetallic Reductive Elimination
3.4.5 Eliminative Reduction
3.5 Insertion
3.5.1 1,2‐Insertion
3.5.2 1,1‐Insertion
3.5.3 Conjugative Insertion
3.5.4 Outer‐Sphere Insertion
3.6 Elimination
3.6.1 β‐Elimination
3.6.2 α‐Elimination
3.7 Transmetallation
3.7.1 Concerted Ring‐Type Transmetallation
3.7.2 Transmetallation Through Electrophilic Substitution
3.7.3 Stepwise Transmetallation
3.8 Metathesis
3.8.1 σ‐Bond Metathesis
3.8.2 Olefin Metathesis
3.8.3 Alkyne Metathesis
References
Part II On the Mechanism of Transition‐metal‐assisted Reactions
4 Theoretical Study of Ni‐Catalysis
4.1 Ni‐Mediated C—H Bond Activation
4.1.1 Ni‐Mediated Arene C—H Activation
4.1.2 Ni‐Mediated Aldehyde C—H Activation
4.2 Ni‐Mediated C—Halogen Bond Cleavage
4.2.1 Concerted Oxidative Addition of C—Halogen Bond
4.2.2 Radical‐Type Substitution of C—Halogen Bond
4.2.3 C—Halogen Bond Cleavage by β‐Halide Elimination
4.2.4 Nucleophilic Substitution of C—Halogen Bond
4.3 Ni‐Mediated C—O Bond Activation
4.3.1 Ether C—O Bond Activation
4.3.2 Ester C—O Bond Activation
4.4 Ni‐Mediated C—N Bond Cleavage
4.5 Ni‐Mediated C—C Bond Cleavage. 4.5.1 C—C Single Bond Activation
4.5.2 C=C Double Bond Activation
4.6 Ni‐Mediated Unsaturated Bond Activation
4.6.1 Oxidative Cyclization with Unsaturated Bonds
4.6.2 Electrophilic Addition of Unsaturated Bonds
4.6.3 Unsaturated Compounds Insertion
4.6.4 Nucleophilic Addition of Unsaturated Bonds
4.7 Ni‐Mediated Cyclization
4.7.1 Ni‐Mediated Cycloadditions
4.7.2 Ni‐Mediated Ring Substitutions
4.7.3 Ni‐Mediated Ring Extensions
References
5 Theoretical Study of Pd‐Catalysis
5.1 Pd‐Catalyzed Cross‐coupling Reactions
5.1.1 Suzuki–Miyaura Coupling
5.1.2 Negishi Coupling
5.1.3 Stille Coupling
5.1.4 Hiyama Coupling
5.1.5 Heck–Mizoroki Reaction
5.2 Pd‐Mediated C—Hetero Bond Formation
5.2.1 C—B Bond Formation
5.2.2 C—S Bond Formation
5.2.3 C—I Bond Formation
5.2.4 C—Si Bond Formation
5.3 Pd‐Mediated C—H Activation Reactions
5.3.1 Chelation‐Free C(sp3)—H Activation
5.3.2 Chelation‐Free C(sp2)—H Activation
5.3.3 Coordinative Chelation‐Assisted ortho‐ C(aryl)—H Activation
5.3.4 Covalent Chelation‐Assisted ortho‐ C(aryl)—H Activation
5.3.5 Chelation‐Assisted meta‐ C(aryl)—H Activation
5.3.6 Coordinative Chelation‐Assisted C(sp3)—H Activation
5.3.7 Covalent Chelation‐Assisted C(sp3)—H Activation
5.3.8 C—H Bond Activation Through Electrophilic Deprotonation
5.3.9 C—H Bond Activation Through σ‐Complex‐Assisted Metathesis
5.3.10 C—H Bond Activation Through Oxidative Addition
5.4 Pd‐Mediated Activation of Unsaturated Molecules
5.4.1 Alkene Activation
5.4.2 Alkyne Activation
5.4.3 Enyne Activation
5.4.4 Imine Activation
5.4.5 CO Activation
5.4.6 Isocyanide Activation
5.4.7 Carbene Activation
5.5 Allylic Pd Complex
5.5.1 Formation from Allylic Oxidative Addition
5.5.2 Formation from Allylic Nucleophilic Substitution
5.5.3 Formation from the Nucleophilic Attack onto Allene
5.5.4 Formation from Allylic C—H Activation
5.5.5 Formation from Allene Insertion
References
6 Theoretical Study of Pt‐Catalysis
6.1 Mechanism of Pt‐Catalyzed C—H Activation
6.1.1 Oxidative Addition of C—H Bond
6.1.2 Electrophilic Dehydrogenation
6.1.3 Carbene Insertion into C—H Bonds
6.2 Mechanism of Pt‐Catalyzed Alkyne Activation
6.2.1 Nucleophilic Additions
6.2.2 Cyclopropanation
6.2.3 Oxidative Cycloaddition
6.3 Mechanism of Pt‐Catalyzed Alkene Activation
6.3.1 Hydroamination of Alkenes
6.3.2 Hydroformylation of Alkenes
6.3.3 Isomerization of Cyclopropenes
References
7 Theoretical Study of Co‐Catalysis
7.1 Co‐Mediated C—H Bond Activation
7.1.1 Hydroarylation of Alkenes
7.1.2 Hydroarylation of Allenes
7.1.3 Hydroarylation of Alkynes
7.1.4 Hydroarylation of Nitrenoid
7.1.5 Oxidative C—H Alkoxylation
7.2 Co‐Mediated Cycloadditions
7.2.1 Co‐Mediated Pauson–Khand Reaction
7.2.2 Co‐Catalyzed [4+2] Cyclizations
7.2.3 Co‐Catalyzed [2+2+2] Cyclizations
7.2.4 Co‐Catalyzed [2+2] Cyclizations
7.3 Co‐Catalyzed Hydrogenation
7.3.1 Hydrogenation of Carbon Dioxide
7.3.2 Hydrogenation of Alkenes
7.3.3 Hydrogenation of Alkynes
7.4 Co‐Catalyzed Hydroformylation
7.4.1 Direct Hydroformylation by H2 and CO
7.4.2 Transfer Hydroformylation
7.5 Co‐Mediated Carbene Activation
7.5.1 Arylation of Carbene
7.5.2 Carboxylation of Carbene
7.6 Co‐Mediated Nitrene Activation
7.6.1 Aziridination of Olefins
7.6.2 Amination of Isonitriles
7.6.3 Amination of C—H Bonds
References
8 Theoretical Study of Rh‐Catalysis
8.1 Rh‐Mediated C—H Activation Reactions
8.1.1 Rh‐Catalyzed Arylation of C—H Bond
8.1.2 Rh‐Catalyzed Alkylation of C—H Bond
8.1.3 Rh‐Catalyzed Alkenylation of C—H Bond
8.1.4 Rh‐Catalyzed Amination of C—H Bond
8.1.5 Rh‐Catalyzed Halogenation of C—H Bond
8.2 Rh‐Catalyzed C—C Bond Activations and Transformations
8.2.1 Strain‐driven Oxidative Addition
8.2.2 The Carbon—Cyano Bond Activation
8.2.3 β‐Carbon Elimination
8.3 Rh‐Mediated C—Hetero Bond Activations
8.3.1 C—N Bond Activation
8.3.2 C—O Bond Activation
8.4 Rh‐Catalyzed Alkene Functionalizations
8.4.1 Hydrogenation of Alkene
8.4.2 Diboration of Alkene
8.5 Rh‐Catalyzed Alkyne Functionalizations
8.5.1 Hydroacylation of Alkynes
8.5.2 Hydroamination of Alkynes
8.5.3 Hydrothiolation of Alkynes
8.5.4 Hydroacetoxylation of Alkynes
8.6 Rh‐Catalyzed Addition Reactions of Carbonyl Compounds
8.6.1 Hydrogenation of Ketones
8.6.2 Hydrogenation of Carbon Dioxide
8.6.3 Hydroacylation of Ketones
8.7 Rh‐Catalyzed Carbene Transformations
8.7.1 Carbene Insertion into C—H Bonds
8.7.2 Arylation of Carbenes
8.7.3 Cyclopropanation of Carbenes
8.7.4 Cyclopropenation of Carbenes
8.8 Rh‐Catalyzed Nitrene Transformations
8.8.1 Nitrene Insertion into C—H Bonds
8.8.2 Aziridination of Nitrenes
8.9 Rh‐Catalyzed Cycloadditions
8.9.1 (3+2) Cycloadditions
8.9.2 Pauson–Khand‐type (2+2+1) Cycloadditions
8.9.3 (5+2) Cycloadditions
8.9.4 (5+2+1) Cycloadditions
References
9 Theoretical Study of Ir‐Catalysis
9.1 Ir‐Catalyzed Hydrogenations
9.1.1 Hydrogenation of Alkenes
9.1.2 Hydrogenation of Carbonyl Compounds
9.1.3 Hydrogenation of Imines
9.1.4 Hydrogenation of Quinolines
9.2 Ir‐Catalyzed Hydrofunctionalizations
9.2.1 Ir‐Catalyzed Hydroaminations
9.2.2 Ir‐Catalyzed Hydroarylations
9.2.3 Ir‐Catalyzed Hydrosilylations
9.3 Ir‐Catalyzed Borylations
9.3.1 Borylation of Alkanes
9.3.2 Borylation of Arenes
9.4 Ir‐Catalyzed Aminations
9.4.1 Amination of Alcohols
9.4.2 Amination of Arenes
9.5 Ir‐Catalyzed C—C Bond Coupling Reactions
References
10 Theoretical Study of Fe‐Catalysis
10.1 Fe‐Mediated Oxidations
10.1.1 Alkane Oxidations
10.1.2 Arene Oxidations
10.1.3 Alkene Oxidations
10.1.4 Oxidative Catechol Ring Cleavage
10.2 Fe‐Mediated Hydrogenations
10.2.1 Hydrogenation of Alkenes
10.2.2 Hydrogenation of Carbonyls
10.2.3 Hydrogenation of Imines
10.2.4 Hydrogenation of Carbon Dioxide
10.3 Fe‐Mediated Hydrofunctionalizations
10.3.1 Hydrosilylation of Ketones
10.3.2 Hydroamination of Allenes
10.4 Fe‐Mediated Dehydrogenations
10.4.1 Dehydrogenation of Alcohols
10.4.2 Dehydrogenation of Formaldehyde
10.4.3 Dehydrogenation of Formic Acid
10.4.4 Dehydrogenation of Ammonia‐Borane
10.5 Fe‐Catalyzed Coupling Reactions
10.5.1 C—C Cross‐Couplings with Aryl Halide
10.5.2 C—N Cross‐Couplings with Aryl Halide
10.5.3 C—C Cross‐Couplings with Alkyl Halide
10.5.4 Iron‐Mediated Oxidative Coupling
References
11 Theoretical Study of Ru‐Catalysis
11.1 Ru‐Mediated C—H Bond Activation
11.1.1 Mechanism of the Ru‐Mediated C—H Bond Cleavage
11.1.2 Ru‐Catalyzed C—H Bond Arylation
11.1.3 Ru‐Catalyzed ortho‐Alkylation of Arenes
11.1.4 Ru‐Catalyzed ortho‐Alkenylation of Arenes
11.2 Ru‐Catalyzed Hydrogenations
11.2.1 Hydrogenation of Alkenes
11.2.2 Hydrogenation of Carbonyls
11.2.3 Hydrogenation of Esters
11.2.4 Hydrodefluorination of Fluoroarenes
11.3 Ru‐Catalyzed Hydrofunctionalizations
11.3.1 Hydroacylations
11.3.2 Hydrocarboxylations
11.3.3 Hydroborations
11.4 Ru‐Mediated Dehydrogenations
11.4.1 Dehydrogenation of Alcohols
11.4.2 Dehydrogenation of Formaldehyde
11.4.3 Dehydrogenation of Formic Acid
11.5 Ru‐Catalyzed Cycloadditions
11.5.1 Ru‐Mediated (2+2+2) Cycloadditions
11.5.2 Ru‐Mediated Pauson–Khand Type (2+2+1) Cycloadditions
11.5.3 Ru‐Mediated Click Reactions
11.6 Ru‐Mediated Metathesis
11.6.1 Ru‐Mediated Intermolecular Olefin Metathesis
11.6.2 Ru‐Mediated Intramolecular Diene Metathesis
11.6.3 Ru‐Mediated Alkyne Metathesis
References
12 Theoretical Study of Mn‐Catalysis
12.1 Mn‐Mediated Oxidation of Alkanes
12.1.1 C—H Hydroxylations
12.1.2 C—H Halogenations
12.1.3 C—H Azidations
12.1.4 C—H Isocyanations
12.2 Mn‐Mediated C—H Activations
12.2.1 Electrophilic Deprotonation
12.2.2 σ‐Complex‐Assisted Metathesis
12.2.3 Concerted Metalation–Deprotonation
12.3 Mn‐Mediated Hydrogenations
12.3.1 Hydrogenation of Carbon Dioxide
12.3.2 Hydrogenation of Carbonates
12.4 Mn‐Mediated Dehydrogenations
12.4.1 Dehydrogenation of Alcohols
12.4.2 Dehydrogenative Couplings
References
13 Theoretical Study of Cu‐Catalysis
13.1 Cu‐Mediated Ullmann Condensations
13.1.1 C—N Bond Couplings
13.1.2 C—O Bond Couplings
13.1.3 C—F Bond Couplings
13.2 Cu‐Mediated Trifluoromethylations
13.2.1 Trifluoromethylations Through Cross‐Coupling
13.2.2 Trifluoromethylations Through Oxidative Coupling
13.2.3 Radical‐Type Trifluoromethylations
13.3 Cu‐Mediated C—H Activations
13.3.1 C—H Arylations
13.3.2 C—H Aminations
13.3.3 C—H Hydroxylation
13.3.4 C—H Etherifications
13.4 Cu‐Mediated Alkyne Activations
13.4.1 Azide–Alkyne Cycloadditions
13.4.2 Nucleophilic Attack onto Alkynes
13.4.3 Alkynyl Cu Transformations
13.5 Cu‐Mediated Carbene Transformations
13.5.1 [2+1] Cycloadditions with Alkenes
13.5.2 Carbene Insertions
13.5.3 Rearrangement of Carbenes
13.6 Cu‐Mediated Nitrene Transformations
13.6.1 [2+1] Cycloadditions with Alkenes
13.6.2 Amination of Nitrenes
13.6.3 Nitrene Insertions
13.7 Cu‐Catalyzed Hydrofunctionalizations
13.7.1 Hydroborylations
13.7.2 Hydrosilylation
13.7.3 Hydrocarboxylations
13.8 Cu‐Catalyzed Borylations
13.8.1 Borylation of Alkenes
13.8.2 Borylation of Alkynes
13.8.3 Borylation of Carbonyls
References
14 Theoretical Study of Ag‐Catalysis
14.1 Ag‐Mediated Carbene Complex Transformations
14.1.1 Silver–Carbene Formation
14.1.2 Carbene Insertion into C—Cl Bond
14.1.3 Carbene Insertion into O—H Bond
14.1.4 Nucleophilic Attack by Carbonyl Groups
14.1.5 Carbene Insertion into C—H Bond
14.2 Ag‐Mediated Nitrene Transformations
14.2.1 Silver–Nitrene Complex Formation
14.2.2 Nucleophilic Attack by Unsaturated Bonds
14.2.3 Nucleophilic Attack by Amines
14.3 Ag‐Mediated Silylene Transformations
14.4 Ag‐Mediated Alkyne Activations
14.4.1 π‐Activation of Alkynes
14.4.2 C—H Activation of Alkynes
References
15 Theoretical Study of Au‐Catalysis
15.1 Au‐Mediated Alkyne Activations
15.1.1 Isomerization of Alkynes
15.1.2 Nucleophilic Attack by Oxygen‐Involved Nucleophiles
15.1.3 Nucleophilic Attack by Nitrogen‐Involved Nucleophiles
15.1.4 Nucleophilic Attack by Arenes
15.2 Au‐mediated Alkene Activations
15.2.1 Nucleophilic Addition of Alkenes
15.2.2 Allylic Substitutions
15.3 Au‐mediated Allene Activations
15.3.1 Hydroamination of Allenes
15.3.2 Hydroalkoxylation of Allenes
15.3.3 Cycloisomerization of Allenyl Ketones
15.4 Au‐mediated Enyne Transformations
15.4.1 1,5‐Enyne Cycloisomerizations
15.4.2 1,6‐Enyne Cycloisomerizations
15.4.3 Allenyne Cycloisomerizations
15.4.4 Conjugative Enyne Cycloisomerizations
References
Index. a
b
c
d
e
f
g
h
i
k
l
m
n
o
p
q
r
s
t
u
v
w
z
WILEY END USER LICENSE AGREEMENT
Отрывок из книги
Yu Lan
The 1960s saw remarkable advances in methods, approximations, and the beginnings of the flowering of computers for chemical calculations. The dawn of the modern hybrid density functional theory in the mid‐1990s, borrowing exact exchange from wavefunction theory, made it possible to begin the quantitative calculations of structure, mechanics, and mechanisms, including the incredibly useful organometallic reactions. Both Ru and Mo catalysts for olefin metathesis, and Pd catalysis for cross‐coupling reactions, have led to Nobel prizes for their discoverers.
.....
Scheme 2.3 The combination of Gaussian‐type orbitals (GTOs) for the construction of Slater‐type orbital (STO). (a) STO. (b) GTO. (c) The combination of GTOs.
Following a suggestion of Boys, Pople decided to use a combination of Gaussian‐type functions to mimic the STO, which was named Gaussian‐type orbital (GTO). These orbitals have a different spatial function, X′Y‴Z′e−ζr2; therefore, the integrals required to build the Fock matrix can be evaluated exactly (Scheme 2.3b). The tradeoff is that GTOs do differ in shape from the STOs, particularly at the nucleus where the STO has a cusp, but the GTO is continually differentiable. The computational advantage is so substantial that it is more efficient to represent a single atomic orbital as a combination of several GTOs rather than a single STO (Scheme 2.3c). When a few GTOs with differing shapes are added, the result is a function that resembles an STO. Now, the basis set is not just the atomic orbitals, but is instead all the GTOs that are used to make up the atomic orbitals. The minimum Gaussian‐type basis set is STO‐3G, in which “STO” is the abbreviation of STO, and “3G” means that each STO is obtained by a linear combination of three GTOs [66, 67].
.....