Chemistry and Biology of Non-canonical Nucleic Acids
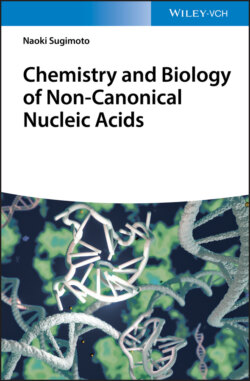
Реклама. ООО «ЛитРес», ИНН: 7719571260.
Оглавление
Naoki Sugimoto. Chemistry and Biology of Non-canonical Nucleic Acids
Table of Contents
List of Tables
List of Illustrations
Guide
Pages
Chemistry and Biology of Non-Canonical Nucleic Acids
Preface
1 History for Canonical and Non-canonical Structures of Nucleic Acids
1.1 Introduction
1.2 History of Duplex
1.3 Non-Watson–Crick Base Pair
1.4 Nucleic Acid Structures Including Non-Watson–Crick Base Pairs
1.5 Perspective of the Research for Non-canonical Nucleic Acid Structures
1.6 Conclusion and Perspective
References
2 Structures of Nucleic Acids Now
2.1 Introduction
2.2 Unusual Base Pairs in a Duplex
2.2.1 Hoogsteen Base Pair
2.2.2 Purine–Pyrimidine Mismatches
2.2.3 Purine–Purine Mismatches
2.2.4 Pyrimidine–Pyrimidine Mismatches
2.3 Non-canonical Backbone Shapes in DNA Duplex
2.4 Branched DNA with Junction
2.5 Multi-stranded DNA Helices
2.6 Structures in RNA
2.6.1 Basic Structure Distinctions of RNA
2.6.2 Elements in RNA Secondary Structures
2.6.2.1 Hairpin Loop
2.6.2.2 Bulge Loop
2.6.2.3 Internal Loop
2.6.3 Elements in Tertiary Interactions of RNA
2.6.3.1 A-Minor Interactions
2.6.3.2 Ribose Zipper
2.6.3.3 T-Loop Motif
2.6.3.4 Kissing-Loop Interaction
2.6.3.5 GNRA Tetraloop Receptor Interaction
2.6.3.6 Pseudoknot Crosslinking Distant Stem Regions
2.7 Conclusion
References
3 Stability of Non-canonical Nucleic Acids
3.1 Introduction
3.2 Factors Influencing Stabilities of the Canonical Duplexes. 3.2.1 Hydrogen Bond Formations
3.2.2 Stacking Interactions
3.2.3 Conformational Entropy
3.3 Thermodynamic Analysis for the Formation of Duplex
3.4 Factors Influencing Stabilities of the Non-canonical Nucleic Acids. 3.4.1 Factors Influencing Stability of Triplexes
3.4.2 Factors Influencing Stability of Quadruplex. 3.4.2.1 G-Quadruplexes
3.4.2.2 i-Motif
3.5 Thermodynamic Analysis for the Non-canonical Nucleic Acids. 3.5.1 Thermodynamic Analysis for the Intramolecular Triplex and Tetraplex
3.5.2 Thermodynamic Analysis for the Intermolecular Triplex
3.5.3 Thermodynamic Analysis for the Tetraplex
3.6 Conclusion
References
4 Physicochemical Properties of Non-canonical Nucleic Acids
4.1 Introduction
4.2 Spectroscopic Properties of Non-canonical Nucleic Acids. 4.2.1 Effect of Non-canonical Structure on UV Absorption
4.2.2 Circular Dichroism of Non-canonical Nucleic Acids
4.2.3 NMR Spectroscopy
4.2.4 Other Spectroscopic Characteristics of Non-canonical Nucleic Acids
4.3.1 Hydration
4.3.2 Cation Binding
4.3.3 pH Effect
4.3.4 Chemical Modification
4.4.1 Specificity of a Ligand to Non-canonical Structures
4.4.2 Fluorescence Platform of Non-canonical Structures
4.4.3 Interface Between Proteins and Nucleic Acids
4.5 Physicochemical Property of Non-canonical Nucleic Acids in Cell. 4.5.1 Molecular Crowding Condition that Reflects Cellular Environments
4.5.2 Effects of Crowding Reagents on Non-canonical Nucleic Acid Structures
4.5.3 Quantification of Physical Properties of Non-canonical Structures in Crowding Condition
4.5.4 Non-canonical Structures Under Mimicking Organelle Environment
4.5.5 Insight for the Formation of Non-canonical Nucleic Acids in Cells
4.6 Conclusion
References
5 Telomere
5.1 Introduction
5.2 Structural Properties of Telomere. 5.2.1 Structures of Telomere
5.2.2 Structural Properties of Human Telomeric G4s
5.2.3 Structure of Repeats of Human Telomeric G4s
5.3 Biological Relevance of Telomere G4. 5.3.1 Telomerase Activity
5.3.2 Telomerase Repeated Amplification Protocol (TRAP) Assay
5.3.3 Alternative Lengthening of Telomere (ALT) Mechanism
5.4 Other Non-canonical Structures Related to Telomere Region. 5.4.1 Telomere i-Motif
5.4.2 Telomere RNA
5.5 Conclusion
References
6 Transcription
6.1 Introduction
6.2 Transcription Process. 6.2.1 Transcription Initiation
6.2.2 Transcription Elongation
6.2.3 Transcription Termination
6.3 Transcription Process Perturbed by Certain Sequences of DNA and RNA
6.4 Transcription Process Perturbed by Non-canonical Structures of DNA and RNA
6.5 Conclusion
References
7 Translation
7.1 Introduction
7.2 RNAs Involved in Translation Machinery
7.3 General Process of Translation
7.3.1 Translation Initiation
7.3.2 Translation Elongation
7.3.3 Translation Termination
7.4 RNA Structures Affecting Translation Reaction
7.4.1 Modulation of Translation Initiation in Prokaryotes
7.4.2 Modulation of Translation Initiation in Eukaryotes
7.4.3 RNA Structures Affecting Translation Elongation
7.4.4 RNA Structures Affecting Translation Termination
7.5 Conclusion
References
8 Replication
8.1 Introduction
8.2 Replication Machineries
8.3 Replication Initiation. 8.3.1 Mechanism of Activation of Replication Origins
8.3.2 Activation Control of Origins by G4s
8.3.3 Control of Timing of Replication Initiation by G4s
8.4 DNA Strand Elongation. 8.4.1 Mechanism of DNA Strand Elongation
8.4.2 Impact of G4 and i-Motif Formations on DNA Strand Synthesis
8.4.3 Relationship Between G4 and Epigenetic Modification
8.4.4 Expansion and Contraction of Replicating Strand Induced by Hairpin Structures
8.5 Termination of Replication
8.6 Chemistry of the Replication and Its Regulation
8.6.1 Cellular Environments
8.6.2 Control of Replication by Chemical Compounds
8.7 Conclusion
References
9 Helicase
9.1 Introduction
9.2 Function and Structure of Helicases
9.3 Unwinding of Non-canonical DNA Structures by Helicases
9.4 G4 Helicases in Gene Expressions
9.5 G4 Helicases in Replication
9.6 G4 Helicases in Telomere Maintenance
9.7 Relation to Diseases by Loss of G4 Helicases
9.8 Insight into Specific Properties of Activities of G4 Helicase Under Cellular Conditions
9.9 Conclusion
References
10 Dynamic Regulation of Biosystems by Nucleic Acids with Non-canonical Structures
10.1 Introduction
10.2 Time Scale of Biological Reactions
10.2.1 Cell Cycle
10.2.2 Central Dogma
10.2.3 Dynamic Structures of Nucleic Acids
10.3 Processes in the Central Dogma Affected by Dynamics of Nucleic Acid Structures
10.3.1 Epigenetic Regulation Caused by Chemical Modification of DNA
10.3.2 Co-transcriptional Formation of Metastable RNA Structures
10.3.3 Co-transcriptional Translation and Transcription Attenuation
10.3.4 Co-transcriptional Ligand Binding and Gene Regulation
10.3.5 Translation Elongation and Co-translational Protein Folding
10.4 Conclusion
References
11 Cancer and Nucleic Acid Structures
11.1 Introduction
11.2 Detail Mechanism of Cancer. 11.2.1 Cancer Incidence
11.2.2 The Relationship Between Genes and Cancer
11.3 Non-canonical Structures of Nucleic Acids in Cancer Cells. 11.3.1 Structural Characteristics of Nucleic Acids in Cancer Cells
11.3.2 Non-canonical Structures Perturb Gene Expression of Cancer-Related Genes
11.4 Roles of Non-canonical Structures of Nucleic Acids in Cancer Cells. 11.4.1 Monitoring of Non-canonical Structures in Cancer Cells
11.4.2 Regulation of Gene Expressions by the Non-canonical Structures in Cancer Cells
11.5 Conclusion
References
12 Neurodegenerative Diseases and Nucleic Acid Structures
12.1 Introduction
12.2 Protein Aggregation-Induced Neurodegenerative Diseases
12.3 DNA Shows Key Role for Neurodegenerative Diseases
12.4 RNA Toxic Plays a Key Role for Neurological Diseases
12.5 Conclusion
References
13 Therapeutic Applications
13.1 Introduction
13.2 Oligonucleotide Therapeutics
13.2.1 Antisense Oligonucleotide
13.2.2 Functions of Antisense Oligonucleotide Therapeutics
13.2.3 Chemical Modifications in Therapeutic Oligonucleotides
13.2.3.1 Backbone Modified Oligonucleotides
13.2.3.2 Ribose Modified Oligonucleotides
13.2.3.3 Oligonucleotides with Unnatural Backbone
13.2.4 Oligonucleotide Therapeutics Other Than Antisense Oligonucleotide
13.2.4.1 Oligonucleotide Therapeutics Functioning Through RNA Interference
13.2.4.2 Oligonucleotide Therapeutics Functioning Through Binding to Protein
13.3 Non-canonical Nucleic Acid Structures as Therapeutic Targets
13.3.1 Traditional Antibiotics Targeting Structured Region of RNAs
13.3.2 Strategies for Constructing Therapeutic Materials Targeting Structured Nucleic Acids
13.4 Non-canonical Nucleic Acid Materials for Inducing Non-canonical Structures
13.5 Conclusion
References
14 Materials Science and Nanotechnology of Nucleic Acids
14.1 Introduction
14.2 Non-canonical Structure-Based Nanomaterials Resembling Protein Functions. 14.2.1 Aptamer
14.2.2 DNAzyme
14.2.3 Ion Channel
14.3 Protein Engineering Using G4-Binding Protein
14.4 Regulation of Gene Expression by G4-Inducing Materials
14.5 Environmental Sensing
14.5.1 Sensing Temperature in Cells
14.5.2 Sensing pH in Cells
14.5.3 Sensing K+ Ion in Cells
14.5.4 Sensing Crowding Condition in Cells
14.6 Conclusion
References
15 Future Outlook for Chemistry and Biology of Non-canonical Nucleic Acids
15.1 Introduction
15.2 Exploring Potential: Properties of Non-canonical Structures in Unusual Media
15.3 Systemizing Properties: Prediction of the Formation of Non-canonical Nucleic Acids Structures
15.4 Advancing Technology: Applications of Non-canonical Structures Taking Concurrent Reactions into Account
15.4.1 Co-transcriptional Dynamics of G-Quadruplex
15.4.2 Co-transcriptional Functionalization of Riboswitch-Like Sensor
15.4.3 Co-transcriptional RNA Capturing for Selection of Functional RNAs
15.5 Conclusion
References
Index. a
b
c
d
e
f
g
h
i
j
k
l
m
n
o
p
q
r
s
t
u
v
w
x
y
z
WILEY END USER LICENSE AGREEMENT
Отрывок из книги
Naoki Sugimoto
As I mentioned in the Introduction in Chapter 1, there is close to 70 years history in nucleic acid research after the discovery of the double helix DNA structure (B-form) as the canonical one by James Dewey Watson and Francis Harry Compton Crick in 1953 and chemical biology of nucleic acids are facing to new aspect today, that is, non-canonical nucleic acids. Through this book, I expect that readers understand how uncommon structure of nucleic acids became one of the common structures as non-canonical nucleic acids that fascinate us now. This new research field for non-canonical nucleic acids will soon big-spark at the interface of chemistry and biology.
.....
T-loop motif is well-observed motif in the loop–loop interaction regions, which have been identified in a variety of RNAs [30]. T-loop motif generally consists of five consecutive nucleotides assuming a compact U-turn-like loop structure, in which the first and the fifth nucleobases form a base pair irrespective of whether it is canonical Watson–Crick or unusual one. When the T-loop motif is involved in the loop–loop interaction, the fourth and fifth nucleobases in the T-loop sandwich an extra nucleobase, which is derived from separated loop region, to make continuous base stacks. The sandwiched nucleobase usually interacts with the second nucleobase of the T-loop through hydrogen bonding [30]. In crystal structure of phenylalanyl-tRNA derived from Saccharomyces cerevisiae, in which T-loop motif was originally discovered, UUCGA loop of pentanucleotides accommodates guanine nucleobase from different tRNA loop region to form stable tertiary interaction (Figure 2.14).
Kissing-loop interaction is a basic type loop-loop interaction that causes cross-linkage between different helices, which are located in intrastrand or interstrand (Figure 2.15). The basic interaction of the kissing loop is formation of base pairing by complementary sequences in the apical loops of two hairpins. Intramolecular kissing complexes have been found in many RNA structures, ranging from transfer RNA (tRNA), in which length is shorter than 100 nucleotides, to ribosomal RNA (rRNA) with more than 1000 nucleotides [31]. The kissing-loop complex is usually stabilized by coaxial stacking of nucleobases included in the interhelical duplex (Figure 2.15) [32]. On the other hand, even if the sequence in the loop forms only two G·C base pairs without the coaxial stacking, a simplest kissing interaction is observed between hairpins each with a GACG tetraloop [33]. In that case, kissing base pairs are stabilized through cross-strand interactions caused by adjacent adenines in the loop [33].
.....